Abstract
Objectives
To synthesize and characterize different molar weight urethane multimethacrylates with a single stage (one-pot) procedure. To prepare and characterize the properties of related composites.
Methods
Two methacrylate precursors were initially synthesized. Then, these precursors and the multimethacrylate system formed by their coupling were characterized by FTIR and 1 H NMR. The final product was used as a matrix (with TEGDMA and SiO 2 silanized microparticles) in the preparation of composites and their physical and mechanical properties were compared to those of a bis-GMA-based resin. Water sorption and solubility measurements of the composites were also performed.
Results
FTIR and NMR suggested that the proposed synthesis route yields a mixture of mainly urethane-di, -tri, and tetramethacrylates. The composites presented low polymerization shrinkage ( e.g. 1.88 ± 0.08% for a resin with 70% of SiO 2 ) and high flexural strength ( e.g. 124.74 ± 9.68 MPa for a resin with 65% of SiO 2 ) when compared to the bis-GMA based resin and other composites found to date. Water sorption and solubility results show that the composites were deemed compliant with ISO 4049 requirements.
Significance
The mixture containing different molar weight of urethane multimethacrylates showed to be an excellent substitute for bis-GMA, achieving an equilibrium of properties (unlike reports elsewhere which show the enhancement of some parameters in detriment to others) and composites with low polymerization shrinkage, suitable microhardness and degree of conversion, and up to standard water sorption/solubility and flexural strength.
1
Introduction
The history of composite resins of methacrylic monomers began in 1962 with Bowen , when the monomer bisphenol A diglycidyl methacrylate (bis-GMA) was first synthesized. Dental restorative materials often consist of both inorganic filler particles in high loading and an organic matrix. This organic matrix is typically a mixture of dimethacrylate monomers. Besides bis-GMA, other dimethacrylate monomers are used commercially . In fact, bis-GMA is still currently the most widely used monomer in the preparation of dental composites . The characteristics of bis-GMA are high molecular weight and low volatility, high viscosity, fast reaction by free-radical photopolymerization, and excellent final polymer properties, as for example, high mechanical strength . Despite the common use of bis-GMA, some problems exist, especially regarding its high polymerization shrinkage, which leads to the formation of marginal infiltration when used in dental composites. Moreover, the high viscosity of bis-GMA makes inserting inorganic particles and handling more difficult. To solve this difficulty, diluent monomers are used; however, as a consequence, the polymerization shrinkage increases and the flexural strength decreases due to the poorer properties of the diluents, such as occurs when triethylene glycol dimethacrylate (TEGDMA) is used.
In an attempt to solve the bis-GMA drawbacks, various monomers have been developed to substitute bis-GMA. However, the most recent research and development efforts that have addressed polymerization shrinkage reduction show that the issue is still a major concern . Recent review reports have also pointed to leaching and bio-degradation issues, which have led to a tendency to explore the synthesis of derivative monomers from natural resources . Among the group of monomers used in the attempt to reduce polymerization shrinkage, monomers having multiple pendant methacrylate groups, which can lead to the formation of a dendritic structure after polymerization, also called hyperbranched polymers , are very promising due to their high molecular weight, low number of methacrylate groups per volume unit and the high reactivity of the methacrylate groups.
The purpose of the present research was the synthesis of a novel multimethacrylate system and the step-by-step investigation of the chemical structure of the products through FTIR and 1 H NMR. The synthesis product presented a high refractive index, which in blends with diluent monomers leads to a better match with the refractive index of common fillers. It was then used to fabricate composites with SiO 2 microparticles, which were submitted to several tests, such as determination of the degree of conversion, polymerization shrinkage, microhardness, flexural strength, and water sorption and solubility. The results indicate that the new system has potential to be used in the substitution of bis-GMA.
2
Materials and methods
2.1
Materials
All reagents used in our synthesis were purchased from Sigma–Aldrich Inc.: bis[4-(glycidyloxy)phenyl]methane (BGPM, 100%), methacrylic acid (MA, 99%), N,N-dimethylbenzylamine (DBA, 99%), p -hydroxyanisole (pHA, 98%), 2-hydroxyethylmethacrylate (HEMA, 99%), and 4,4′-methylenebis(phenylisocyanate) (MPI, 98%). The commercial monomers used to prepare the composites were bisphenol-A-diglycidylmethacrylate (bis-GMA) and triethyleneglycoldimethacrylate (TEGDMA, 95%). The photoinitiator system was camphorquinone (CQ, 97%) and 2-(N,N-dimethylamino)ethyl methacrylate (DMAEMA, 99%). The inorganic portion was silica (V258 2507, SP 345, 5% silane, 0.7 μm, density 3.0 g/mL, refractive index 1.541, Esstech Inc., Essington, USA), unless otherwise noted. All the chemicals in this study were used as received, except for methanol, which was double distilled and kept dried using molecular sieves. The synthesis was performed in two major steps: (i) the synthesis of the methacryloyloxypropylphenylmethanes (BMPMs) and the urethane-methacryloyloxyethyl (UMEs) precursors and (ii) the urethane-multimethacrylate mixture (referred to hereafter just as M mixture).
2.2
Methods
1 H NMR experiments were carried out using a Varian Mercury Plus BB, operating at 300 MHz with a 5 mm probe for liquids (ATB) and field gradient. To obtain the spectra, the samples (10 mg) were dissolved in 0.7 mL of CDCl 3 . The acquisition parameters were as follows: a pulse of 45°, a recycle delay of 1 second, and acquisition of 32 transients. Zero-filling and time-domain data apodized with a 0.2 Hz (lb) exponential function were applied to improve signal-to-noise ratio before the Fourier transform. These experiments were performed using tetramethylsilane as a reference.
For the synthesis of precursor 1 (BMPMs), 0.5 mol of BGPM was placed in a 100-mL flask equipped with a magnetic stirrer and a dropping funnel, which was immersed in a thermobath for temperature control. Next, 0.6 wt% of N,N-dimethylbenzylamine (DBA) and 0.3 wt% of p -hydroxyanisole ( p -HA) were also placed in the flask, and then the mixture was stirred and heated. Methacrylic acid (1.0 mol) was added dropwise to the mixture at 70–80 °C. After the addition of methacrylic acid, the reaction was kept at 105 °C for 6 h. As discussed with details in advance, this reaction yields a mixture of isomers, Fig. 1 A , (A) bis[4-(2-hydroxy-3-methacryloyloxypropyl)phenyl]methane, (B) 4-(2-hydroxy-3-methacryloyloxypropyl)-4′-(2-methyleneoxy-2-methacryloyloxypropyl)-(bisphenyl)methane, and (C) bis[4-(2-methyleneoxy-2-methacryloyloxypropyl)phenyl]methane (BMPMs).
In the synthesis of precursor 2 (UMEs, Fig. 1 B), 1.0 mol of MPI was placed in a 100-mL flask and continuously purged with dry nitrogen to remove oxygen and moisture. Soon afterwards, 1.0 mol of HEMA was added dropwise to the flask. The reaction temperature was kept at 35 °C while the mixture was vigorously stirred for 4 h; no purification procedures were used at the end. An FTIR spectrometer was used to monitor the changes in the intensity of the isocyanate group signal (NCO, 2295 cm −1 relative to the aromatic signal at ca. 1600 cm −1 ). This reaction was expected to yield mainly monosubstituted MPI (4-(2-methacryloyloxyethoxycarbonylamino)-4′-isocyanate-(bisphenyl)methane) and disubstituted MPI (4,4′-bis-[2-methacryloyloxyethoxycarbonylamino)phenyl]methane). The scheme of the synthesis is shown in Fig. 1 B.
In order to synthesize the urethane-multimethacrylates from precursors 1 and 2, a pre-determined mass of 5.1 g of BMPM was dissolved in pure acetone and then 8.0 g of the urethane methacrylate mixture (UME + MPI) were added to the reaction mixture. The temperature was kept at 40 °C under nitrogen atmosphere for 4 h and no purification procedures were used at the end. The viscosity of the M mixture, as well as of the bis-GMA, was measured using a Cannon-Ubbelohde SMI-micro dilution-type viscometer at 35 °C. The refractive indices were measured using an Atago RX 5000 Alpha refractometer at 25 °C.
To prepare the composites, the M mixture and the diluent monomer TEGDMA were dissolved in acetone, and then silica microparticles were added. After stirring for 10 min, the initiator systems, DMAEMA (1%, wt%) and CQ (1%, wt%), were added, and the mixture was stirred in a darkroom and open system for 24 h. Then, the remaining solvent was removed from the paste under vacuum at 40 °C for another 24 h. The compositions of the resins are listed in Tables 1 and 2 , and there are two composites with different composition (composites 5 and 6 in Table 2 ), where the inorganic portion was divided in 85% of 0.7 μm silanized Ba glass and 15% of OX-50 (average particle size 50 nm), both from ESSTECH. Curing was performed by exposing the samples to 470 nm visible light (Radii-e unit, SDI/Australia, 1200 mW/cm 2 ) for 60 s (samples used to measure degree of conversion and polymerization shrinkage), 120 s for hardness, and three overlapping exposures of 60 s for samples used in flexural and sorption/solubility tests, following ISO 4049. The DC (degree of conversion, %) measurements were performed as follows: disk-shaped specimens (0.5 mm thick) were molded between glass slides and subsequently subjected to near-IR (NIR) spectroscopy (Nexus 6700 IR Spectrometer, Thermo Scientific, Waltham, MA) in transmission mode. The integrated peak area corresponding to the 6165 cm −1 methacrylate vinyl first overtone collected before and after polymerization was used to calculate DC. The microhardness was measured with an HVS-5 Vicker’s indenter (0.2 kgf load for 20 s) in 1.0 mm thick and 5.0 mm in diameter cured samples (curing each face with three overlapping 60 s exposures, protected by a polyester thin film). The water sorption and solubility tests, as well as the flexural strength assays, were carried out according to standard ISO 4049, which has not been reproduced here. The flexural strength measurements were performed in an LK10 universal testing machine (Lloyd Instruments ltd.). Volumetric shrinkage (VS) for each composite ( n = 3) was determined in a mercury dilatometer (ADA Health Foundation, Gaithersburg, MD, USA) composite samples of 150 mg were placed on glass slides that had been sandblasted with aluminum oxide (150 μm) and coated with a silane coupling agent (Ceramic Primer, 3 M ESPE, St. Paul, MN, USA). The glass slide was clamped to the dilatometer column, on top of which a LVDT (linear variable differential transducer) was placed in contact with the surface of the mercury. The composite was photoactivated through the glass slide for 60 s. LVDT readings recorded for 60 min at room temperature were converted into volumetric shrinkage, based on previously input data of composite mass and density (determined by the Archimedes method). The thermal expansion effect produced by the heat generated from the curing light was subtracted from the results by performing a second 30 s irradiation after 60 min of data acquisition, and following the volumetric change for 30 min.
Sample | Composition | Hardness [HV] | FS [MPa] | Sorp./Solub. [μg/mm 3 ] |
---|---|---|---|---|
1 | (bis-GMA 50 TEG 50 ) 40 Si 60 | 35.96 (0.26) | 88.13 (7.89) | 34.1 (1.3)/0.650 (0.021) |
2 | (M 50 TEG 50 ) 40 Si 60 | 44.22 (0.42) | 108.30 (4.33) | 33.6 (2.8)/0.573 (0.014) |
3 | (M 50 TEG 50 ) 35 Si 65 | 48.68 (0.35) | 124.74 (9.68) | 30.5 (1.7)/0.492 (0.013) |
4 | (M 50 TEG 50 ) 30 Si 70 | 62.06 (0.22) | 110.42 (4.63) | 28.3 (0.8)/0.350 (0.021) |
Sample | Composition | ΔVp [%] | DC [%] |
---|---|---|---|
1 | (bis-GMA 50 TEG 50 ) 40 Si 60 | – | 75.78 (0.42) |
2 | (M 50 TEG 50 ) 40 Si 60 | 2.82 (0.74) | 77.43 (0.76) |
3 | (M 50 TEG 50 ) 35 Si 65 | 2.99 (0.62) | 77.99 (0.85) |
4 | (M 50 TEG 50 ) 30 Si 70 | 3.18 (0.72) | 76.63 (0.16) |
5 | (M 70 TEG 30 ) 30 Si 70 | 1.88 (0.08) | 71.47 (0.93) |
6 | (bis-GMA 70 TEG 30 ) 30 Si 70 | 4.34 (0.01) | 72.19 (1.85) |
2
Materials and methods
2.1
Materials
All reagents used in our synthesis were purchased from Sigma–Aldrich Inc.: bis[4-(glycidyloxy)phenyl]methane (BGPM, 100%), methacrylic acid (MA, 99%), N,N-dimethylbenzylamine (DBA, 99%), p -hydroxyanisole (pHA, 98%), 2-hydroxyethylmethacrylate (HEMA, 99%), and 4,4′-methylenebis(phenylisocyanate) (MPI, 98%). The commercial monomers used to prepare the composites were bisphenol-A-diglycidylmethacrylate (bis-GMA) and triethyleneglycoldimethacrylate (TEGDMA, 95%). The photoinitiator system was camphorquinone (CQ, 97%) and 2-(N,N-dimethylamino)ethyl methacrylate (DMAEMA, 99%). The inorganic portion was silica (V258 2507, SP 345, 5% silane, 0.7 μm, density 3.0 g/mL, refractive index 1.541, Esstech Inc., Essington, USA), unless otherwise noted. All the chemicals in this study were used as received, except for methanol, which was double distilled and kept dried using molecular sieves. The synthesis was performed in two major steps: (i) the synthesis of the methacryloyloxypropylphenylmethanes (BMPMs) and the urethane-methacryloyloxyethyl (UMEs) precursors and (ii) the urethane-multimethacrylate mixture (referred to hereafter just as M mixture).
2.2
Methods
1 H NMR experiments were carried out using a Varian Mercury Plus BB, operating at 300 MHz with a 5 mm probe for liquids (ATB) and field gradient. To obtain the spectra, the samples (10 mg) were dissolved in 0.7 mL of CDCl 3 . The acquisition parameters were as follows: a pulse of 45°, a recycle delay of 1 second, and acquisition of 32 transients. Zero-filling and time-domain data apodized with a 0.2 Hz (lb) exponential function were applied to improve signal-to-noise ratio before the Fourier transform. These experiments were performed using tetramethylsilane as a reference.
For the synthesis of precursor 1 (BMPMs), 0.5 mol of BGPM was placed in a 100-mL flask equipped with a magnetic stirrer and a dropping funnel, which was immersed in a thermobath for temperature control. Next, 0.6 wt% of N,N-dimethylbenzylamine (DBA) and 0.3 wt% of p -hydroxyanisole ( p -HA) were also placed in the flask, and then the mixture was stirred and heated. Methacrylic acid (1.0 mol) was added dropwise to the mixture at 70–80 °C. After the addition of methacrylic acid, the reaction was kept at 105 °C for 6 h. As discussed with details in advance, this reaction yields a mixture of isomers, Fig. 1 A , (A) bis[4-(2-hydroxy-3-methacryloyloxypropyl)phenyl]methane, (B) 4-(2-hydroxy-3-methacryloyloxypropyl)-4′-(2-methyleneoxy-2-methacryloyloxypropyl)-(bisphenyl)methane, and (C) bis[4-(2-methyleneoxy-2-methacryloyloxypropyl)phenyl]methane (BMPMs).
In the synthesis of precursor 2 (UMEs, Fig. 1 B), 1.0 mol of MPI was placed in a 100-mL flask and continuously purged with dry nitrogen to remove oxygen and moisture. Soon afterwards, 1.0 mol of HEMA was added dropwise to the flask. The reaction temperature was kept at 35 °C while the mixture was vigorously stirred for 4 h; no purification procedures were used at the end. An FTIR spectrometer was used to monitor the changes in the intensity of the isocyanate group signal (NCO, 2295 cm −1 relative to the aromatic signal at ca. 1600 cm −1 ). This reaction was expected to yield mainly monosubstituted MPI (4-(2-methacryloyloxyethoxycarbonylamino)-4′-isocyanate-(bisphenyl)methane) and disubstituted MPI (4,4′-bis-[2-methacryloyloxyethoxycarbonylamino)phenyl]methane). The scheme of the synthesis is shown in Fig. 1 B.
In order to synthesize the urethane-multimethacrylates from precursors 1 and 2, a pre-determined mass of 5.1 g of BMPM was dissolved in pure acetone and then 8.0 g of the urethane methacrylate mixture (UME + MPI) were added to the reaction mixture. The temperature was kept at 40 °C under nitrogen atmosphere for 4 h and no purification procedures were used at the end. The viscosity of the M mixture, as well as of the bis-GMA, was measured using a Cannon-Ubbelohde SMI-micro dilution-type viscometer at 35 °C. The refractive indices were measured using an Atago RX 5000 Alpha refractometer at 25 °C.
To prepare the composites, the M mixture and the diluent monomer TEGDMA were dissolved in acetone, and then silica microparticles were added. After stirring for 10 min, the initiator systems, DMAEMA (1%, wt%) and CQ (1%, wt%), were added, and the mixture was stirred in a darkroom and open system for 24 h. Then, the remaining solvent was removed from the paste under vacuum at 40 °C for another 24 h. The compositions of the resins are listed in Tables 1 and 2 , and there are two composites with different composition (composites 5 and 6 in Table 2 ), where the inorganic portion was divided in 85% of 0.7 μm silanized Ba glass and 15% of OX-50 (average particle size 50 nm), both from ESSTECH. Curing was performed by exposing the samples to 470 nm visible light (Radii-e unit, SDI/Australia, 1200 mW/cm 2 ) for 60 s (samples used to measure degree of conversion and polymerization shrinkage), 120 s for hardness, and three overlapping exposures of 60 s for samples used in flexural and sorption/solubility tests, following ISO 4049. The DC (degree of conversion, %) measurements were performed as follows: disk-shaped specimens (0.5 mm thick) were molded between glass slides and subsequently subjected to near-IR (NIR) spectroscopy (Nexus 6700 IR Spectrometer, Thermo Scientific, Waltham, MA) in transmission mode. The integrated peak area corresponding to the 6165 cm −1 methacrylate vinyl first overtone collected before and after polymerization was used to calculate DC. The microhardness was measured with an HVS-5 Vicker’s indenter (0.2 kgf load for 20 s) in 1.0 mm thick and 5.0 mm in diameter cured samples (curing each face with three overlapping 60 s exposures, protected by a polyester thin film). The water sorption and solubility tests, as well as the flexural strength assays, were carried out according to standard ISO 4049, which has not been reproduced here. The flexural strength measurements were performed in an LK10 universal testing machine (Lloyd Instruments ltd.). Volumetric shrinkage (VS) for each composite ( n = 3) was determined in a mercury dilatometer (ADA Health Foundation, Gaithersburg, MD, USA) composite samples of 150 mg were placed on glass slides that had been sandblasted with aluminum oxide (150 μm) and coated with a silane coupling agent (Ceramic Primer, 3 M ESPE, St. Paul, MN, USA). The glass slide was clamped to the dilatometer column, on top of which a LVDT (linear variable differential transducer) was placed in contact with the surface of the mercury. The composite was photoactivated through the glass slide for 60 s. LVDT readings recorded for 60 min at room temperature were converted into volumetric shrinkage, based on previously input data of composite mass and density (determined by the Archimedes method). The thermal expansion effect produced by the heat generated from the curing light was subtracted from the results by performing a second 30 s irradiation after 60 min of data acquisition, and following the volumetric change for 30 min.
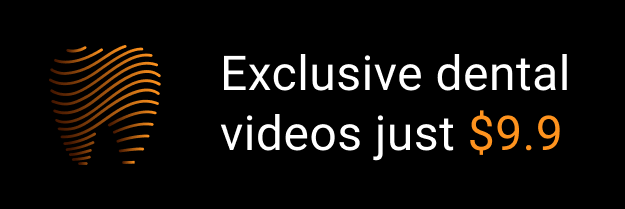