Abstract
Objectives
The aim of this study was to investigate if a synthetic granular calcium phosphate compound (CPC) and a composite bisphosphonate-linked hyaluronic acid–calcium phosphate hydrogel (HABP·CaP) induced similar or more amount of bone as bovine mineral in a modified sinus lift rabbit model.
Material and methods
Eighteen adult male New Zeeland White rabbits, received randomly one of the two test materials on a random side of the face, and bovine mineral as control on the contralateral side. In a sinus lift, the sinus mucosa was elevated and a titanium mini-implant was placed in the alveolar bone. Augmentation material (CPC, HABP·CaP or bovine bone) was applied in the space around the implant. The rabbits were euthanized three months after surgery and qualitative and histomorphometric evaluation were conducted. Histomorphometric evaluation included three different regions of interest (ROIs) and the bone to implant contact on each installed implant.
Results
Qualitative assessment (p = <.05), histomorphometric evaluations (p = < .01), and implant incorporation (p = <.05) showed that CPC and bovine mineral induced similar amount of bone and more than the HABP·CaP hydrogel.
Conclusion
CPC induced similar amount of bone as bovine mineral and both materials induced more bone than HABP·CaP hydrogel.
Clinical significance
The CPC is suggested as a synthetic alternative for augmentations in the maxillofacial area.
1
Introduction
Autologous bone grafting is used for augmentation in atrophic edentulous jaw areas but is suboptimal because of unpredictable resorption of the graft. To reduce patient morbidity, there is a need for new replacement strategies with osteoconductive and osteoinductive materials. There are several materials already on the market, which are osteoconductive and are used successfully for this purpose [ ]. Bovine bone mineral (hydroxyapatite) has been extensively explored during the past decades in connection with implant placement [ , ]. Bovine mineral is reported to be biocompatible and a well-functioning space maintainer but not resorbable at least during an 11-year timeframe [ ].
Since the 1980s, various synthetic calcium phosphate based materials have been in focus of research regarding biocompatibility and other properties, including osteoconductivity. Lately there has been a considerable interest in different injectable gels for tissue repair such as hyaluronan gels combined with different bone inducing substances [ ]. Bone inducing substitute materials are advantageous over autologous bone grafts since graft site morbidity and severe patient concerns such as pain, postoperative bleeding, superficial skin sensory impairment, functional disorders ( e.g. disturbed gait) are avoided [ ]. Further, replacing the bone substitute materials derived from animal origin eliminates the risk of zoonotic infections and results in less ethical, cultural, and religious considerations for the patients. An optimal bone substitute material should reduce grafting site morbidity, induce osteoconductivity or/and osteoinductivity, and ultimately keep a balance between volume and resorption working as a scaffold.
Studies show that several of the calcium phosphate materials have abilities for bone conduction, bone induction, and resorption of the material itself [ ]. Hydroxyapatite, brushite and monetite are different crystalline phases of calcium phosphate, similar in their chemical construction but presenting quite different properties. Hydroxyapatite is a bone conductive material and shows low, or no resorption rate in vivo [ ] whilst monetite demonstrate both bone inductive and conductive properties as well as resorbability [ ]. Brushite on the other hand, is quite reactive and transforms quickly into hydroxyapatite but can also synthetically be made as monetite. In the presence of pyrophosphate, monetite and brushite have the tendency to stay in their active form until resorbed [ ]. Calcium phosphate resorption is mediated by both a cellular processes and passive dissolution [ ]. Pyrophosphate is produced by hydrolysis of extracellular ATP and is a well-known and important component in bone mineralisation where it acts as an inhibitor and regulator of hydroxyapatite formation [ , , ].
The calcium phosphate compound (CPC) used in this study has been shown to have a slow resorption rate and is now in use as a solid scaffold for large skull defects in humans [ ].
Bisphosphonates (BP) are chemically analogues to pyrophosphates with strong binding capacity to hydroxyapatite. They are used in treatments of osteoporosis and are known to influence bone formation by decreasing osteoclast activity, but also to some degree increase osteoblast activity [ , ]. Nejadnik et al. recently showed that non-covalent cross-linked Bisphosphonate-hyaluronic acid-calcium phosphate nanocomposite hydrogel (HABP·CaP) could heal itself if ruptured. After implantation of this material in rat bone defects, formation of new trabecular-like bone was detected not only at the edges of the defect but also in the middle of the grafted material after 4 weeks [ ]. In the study by Hulsart-Billström et al. it was shown that BPs covalently linked to hyaluronic acid (HA) hydrogel exhibited reduced release rate of the in situ encapsulated bone morphogenic protein (BMP) [ ]. This effect was believed to prolong the local activity of the morphogen, which in combination with anti-resorptive activities of the immobilized BPs should be helpful in increasing bone formation [ , ].
In the present study, the aim was to explore if the granular form of the CPC material is a potentially suitable grafting material in the maxillofacial area e.g. , in sinus augmentations. Further, we wanted to investigate the possible benefits that HABP·CaP may have on local bone formation. The hypothesis was that the two test materials would be as efficient with regards to bone formation as bovine bone in a rabbit sinus lift model.
2
Materials and methods
2.1
Animals and study design
Eighteen adult male New Zeeland White rabbits from a specific-pathogen-free colony (Lidköpings Kaninfarm, Lidköping, Sweden) were used. The breeding colony was free from known rabbit pathogens according to recommended health monitoring [ ] At the time of surgery, the rabbits were 36 ± 4 weeks old and weighed 3.7 ± 0.2 kg (mean ± SD). The rabbits were housed individually in cages with a floor area of 0.42 m 2, equipped with a shelf and a covered area. Standard pelleted rabbit diet (Lactamin K3, Lantmännen, Stockholm, Sweden) and autoclaved hay were fed and rabbits had access to autoclaved straw for bedding and water ad lib . The light – dark cycle was 12:12 h with lights on at 07:00. Room temperature was 18 ± 3° C and humidity 55 + 10%. The animals were acclimatized for two weeks and accustomed to handling. On the day before surgery, the rabbits were clinically examined.
The study was designed as a prospective, randomised, controlled experiment and was approved by the Uppsala ethics committee for animal experiments (C 70/13). The animals were randomised in two groups (Microsoft Office Excel 2007, Microsoft, Kista, Sweden): Group CPC Granule (n = 9) and Group HABP·CaP Granule (n = 9). Furthermore, the side for placement of the test material was randomised to the maxillary sinus of one side and the control material, i.e. bovine mineral (Geistlich Bio-Oss ® , Geistlich Pharma AG, Wolhusen, Switzerland) was placed in contralateral sinus. The rabbits were also subjected to an unrelated study on the effect of the non-steroidal anti-inflammatory drug (NSAID) carprofen on bone formation [ ]. Within each material group, rabbits were randomised to receive either 5 mg/ kg of carprofen (Norocarp vet, 50 mg/ml, N-Vet, Uppsala, Sweden) or the equivalent volume of saline. The protocol ran for three months.
2.2
Material preparation
2.2.1
Calcium phosphate ceramic granules (CPC)
The preparation of the calcium phosphate compound was based on a previously published composition [ ]. The cement was prepared from mono-calcium phosphate monohydrate (MCPM), β-tricalcium phosphate (β-TCP), glycerol and deionized water. 0.4 mL of water and 4.5 g of glycerol were stirred into a homogenous liquid before it was added to a Falcon tube with a mixture of 11.2 g of MCPM and 13.8 g of β-TCP. The cement was first mixed in a cap vibrator (Ivoclar Vivadent AG, Schaan, Liechtenstein) followed by a quick mixing with a mortar and pestle. The cement paste was subsequently extruded through a 1 mm holed screen (Caleva bench-top screen extruder, Caleva process solutions Ltd. UK, Dorset) and shaped into granules (Spheronizer 120, Caleva process solutions Ltd. UK, Dorset).
The granules were sieved to obtain granules of a size between 0.60 mm and 1.18 mm, after which they were cured in 100% humidity at 37 °C for 24 h. The glycerol was removed by dissolution in a water bath for 48 h. The granules were then dried at room temperature for 24 h in a laminar flow cabinet. The final step was to autoclave the granules at 124 °C for 20 min. The phase composition of the granules was characterized by X-ray diffraction (XRD; D8 Advance, Bruker AXS GmbH, Karlsruhe, Germany). The granules were ground by mortar and pestle prior to testing. A structural characterization with a 2-theta setup was used with a beam knife, a Nickel filter and a Cu-K irradiation source. The resulting diffractogram was collected between 2θ of 5–60° at steps of 0.02° with 0.25 s per step and a rotation of 80 rpm. Phase composition of CPC granules from XRD analysis was β-CPP 6.1 wt%, β –TCP 10.4 wt%, Brushite 0.5 wt% and Monetite 82.8 wt%, followed by Rietveld refinement. The different crystalline phases were quantified by Rietveld refinement analysis, using BGMN software ( www.bgmn.de ) with Profex interface ( http://profex.doebelin.org ). The reference structures were PDF#04-008-8714 for β-TCP [ ] PDF#04-009-3876 for brushite [ ]. PDF#04-009-3755 for monetite [ ] and PDF#04-009-3876 for β-calcium pyrophosphate (β-CPP) [ ]. ( Fig. 1 ) All work was performed using sterile technique.

2.2.2
Bisphosphate-hyaluronic acid-calcium phosphate nanocomposite hydrogel (HABP·CaP)
Physically cross-linked HABP·CaP nanocomposite hydrogel material was prepared according to our previously published procedure [ ]. Formation of the nanocomposite hydrogel is triggered by mixing of equal volumes of two aqueous solutions, 4% hyaluronan-bisphosphonate and 12% hydroxyapatite, 1.5 mL of 4% hyaluronan-bisphosphonate solution and 1.5 mL of 12% hydroxyapatite dispersion were taken into two separate 3 mL syringes, which were then connected through a plastic connector ( Fig. 2 ). Mixing of the components was achieved by passing the liquid precursors from one syringe to the other several times. The nanocomposite hydrogel was formed and the nanocomposite finally collected in one of the syringes. The other syringe was taken away and the nanocomposite was extruded through a needle in portions onto a balance plate. Nine portions of approximately 325 mg by weight were made and transferred into Eppendorf tubes.

2.2.3
Deproteinized bovine bone mineral (DBBM)
As control material bovine mineral was chosen and commercially attained (Geistlich, Bio-Oss®, Geistlich Pharma AG, Wolhusen, Switzerland). Particle size was 0.25–1 mm. The characteristics of this biomaterial have been extensively described elsewhere [ , ].
2.2.3.1
Surgery
The surgical procedure was a modification of a previous rabbit animal model by Kim et al. [ ]. The rabbits were premedicated subcutaneously (s.c.) with the sedative medetomidine (2 mg/kg, Domitor ® Orion Pharma Animal Health, Sollentuna, Sweden) and intramuscularly (i.m.). with the antibiotic ceftiofur (5 mg/kg, Exenel ® , Orion Pharma AB, Animal Health, Sollentuna, Sweden). Anaesthesia was induced and maintained by continuous infusion of sufentanil (Sufenta ® , Jansen-Cilag, Sollentuna, Sweden) and midazolam (Midazolam Actavis, Actavis AB, Stockholm, Sweden) as described by Hedenqvist et al. [ ]. After skin preparation of the cheek area (including shaving and washing with 2% Chlorhexidine), 4.5 mg (0.9 mL) of prilocain (Citanest ® , 5 mg/ml, AstraZeneca, Sweden) was administered sc. per side. A skin incision was made in the cheek area a few mm above the inferior border of the incisive bone and the maxilla. The subcutaneous tissue and masseter muscle were divided to expose the periosteum. A bone window (10 mm × 10 mm) was made with piezo-electric surgery (Mectron, Carasco, Italy) in the lateral wall of the maxilla and removed. Great care was taken not to perforate the sinus mucosa and to elevate it carefully. The periosteum was elevated and a miniature dental titanium implant was installed in the edentulous alveolar ridge. The implant was 2.2 mm × 6 mm, made by machined commercially pure titanium Grade 4, and cleaned after fabrication in a series of cleaning steps including detergent, butanol, and ethanol, (Enge Mikroteknik, Vittsjö, Sweden). The fixture site was prepared using a round burr of a diameter of 2.0 mm twist drill after making a mark (Astra Tech system drills, DentsplySirona, Mölndal, Sweden). Space was created in the sinus cavity, under the elevated sinus mucosa, allowing the test material to be applied underneath. The materials (0.5–1.0 mL) were packed as near the titanium implant as possible and the space was filled out until the material reached the bone window. ( Fig. 3 a.b.c.) Over the bone window, a 25 mm × 25 mm collagen membrane (Geistlich Bio-Gide ® , Geistlich Pharma AG, Wolhusen, Switzerland) was placed over the grafted site to hold the grafting material in place. The periosteum and muscle layer were sutured tightly with non-woven Monocryl sutures 5-0 (Ethicon, Johnson & Johnson AB) to secure the membrane in place, followed by skin sutures with the same material. The surgery lasted 60–90 min and after that, the animals were carefully monitored until fully recovered from anaesthesia. Pain relief was administered s.c every 6 h with buprenorphine (0.01–0.05 mg/kg, Temgesic ® , 0.3 mg/ml, RB Pharmaceuticals, Slough, Berkshire, UK) during the first 72 h. Additionally, half of the animals received carprofen, and all animals ceftiofur, daily for four days.

During the first postoperative week, the animals were clinically examined and weighed daily and after that weekly until the end of the study.
2.3
Qualitative and quantitative analyses
Twelve weeks post-surgery, the rabbits were euthanized with pentobarbital (Allfatal ® vet. Omnidea AB, Stockholm, Sweden) i.v. Examination of the implant area was performed by cone beam computer tomograph (CBCT) (3D Accuitomo 170 J. Morita, Irvine, CA, USA) for guidance of the exact excision of the maxillary bone blocks. The parts of the maxilla containing the implants were removed using a band saw (KT-400, Klaukkala, Finland). The surgical area was grossly examined and the oral cavity inspected.
2.3.1
Sample processing
The samples were first immersed in 10% neutral buffered formalin for 30-31 days, followed by rinsing in tap water and subsequent dehydration. They were then subjected to increasing concentrations of ethanol (70% – 100%), pre-infiltrated in diluted resins before infiltration in pure resin and finally embedded in pure resin (Technovit 7200, Kulzer, Hanau, Germany) and polymerized under UV light (Exakt R Apparatebau, Norderstedt, Germany). Non-decalcified cut and ground sections were prepared according to the Donath technique using the ExaktR equipment [ ]. In brief, the cured samples were divided in the water-cooled band-saw and the surface of each block was ground parallel prior to be glued onto a supporting plexi-glass. An initial thick section was prepared which then was ground (using SiC wet grinding papers starting with 800 grit up to 1200 grit papers) in water-cooled grinding equipment. No final polishing was used. The sections were prepared to have a final thickness of about 15 μm and finally the sections were stained with a mixture of toluidine blue and pyronin [ ].
2.3.2
Histological examination
Qualitative histology was performed as previously described by Hedenqvist et al. [ ]. In the present study, we also included the pilot rabbit in all evaluations. In brief, two veterinary pathologists (CL, SE), blinded to treatment evaluated the sections separately and then agreed on a consensus scoring. One section per animal from each side was examined for degree of implant incorporation in bone (rating as good, satisfactory or poor) and graft material in association with bone (yes or no). The degree of implant incorporation was evaluated according to the following criteria: amount of bone surrounding the implant, amount of grooves containing bone and amount of bone in direct contact with the implant. If all three parameters were fulfilled; i.e. the implant was surrounded by moderate or abundant bone, and moderately-extensively incorporated with regard to number of grooves filled with bone and presence of direct bone-implant contact, the incorporation was scored as good. If only one or two of the criteria were adequate the incorporation was satisfactory; otherwise poor. Also, sections were evaluated for inflammatory changes, such as influx of inflammatory cells.
Histomorphometry was performed by one of the authors (ATr) on the same samples as in the qualitative histology evaluation. All samples were photographed in objectives of x1 and x4 with a Nikon E600 light microscope with Nikon DXM 1200 camera (Nikon E600 microscope and Nikon DXM 1200 camera, Nikon Instruments, Melville, NY, USA) connected to a PC. All slides were photographed under same conditions for exposure time, lamp intensity, and camera gain. The measurements and analysis were done in NIS-elements imaging software (NIS-elements Basic Research, Nikon, Tokyo, Japan). White balance adjustments, calibrations, and transformations of the photographs into binary images were performed on each image. The amount of new bone was marked in red, measured and presented as binary area fraction, e.g. (binary area (red))/(the total region of interest (ROI) area) = binary area fraction. The new and old bone was interpreted visually by its colour, where “pale purple” colour was judged as old bone, while new bone had a more “dark purple” appearance.
For the measurements of the new bone formation in contact to the materials, two different ROIs were used:
- a)
A “Free ROI” (objective of x1) where the external border was outlining the bone found in contact with the tested material. The fact that the material in different samples ended up in different areas around the implant resulted in different area sizes and locations of the Free ROIs, 1,0 mm 2 –17,8 mm 2 (mean area 10,8 mm 2 ). ( Fig. 4 a)
Fig. 4 A schematic illustration of the three different regions of interests (ROIs): a) the Free ROI that include whole material area and bone adjacent to it, marked with red border. b) The Rectangular ROI, size area of 3.3 mm × 2.7 mm, placed were most of the augmented material could be observed, and c) the Implant ROI that include the area of five grooves at both sides. The new bone is presented as area percentage of the different ROI areas in the different ROIs. (For interpretation of the references to colour in this figure legend, the reader is referred to the web version of this article.) - b)
“Rectangular ROI” described the bone–material ratio and had consistent size area between the samples of 3.3 mm × 2.7 mm, (8.9 mm 2 ). Due to the material location around test implants the site of the ROI varied in the samples to were most amount of the tested material could be seen, resulting in same area size but different locations in all the samples . ( Fig. 4 b)
Implant healing and incorporation was evaluated by two different measurements, Implant ROI and BIC (bone to implant contact). Objectives of x4 were used for both of these ROIs. c) For “Implant ROI”, the binary area fraction of the new bone formed in five grooves at both sides of the implant was measured, starting from the third thread from the implant head. ( Fig. 4 c) BIC was measured in five implant grooves at both sides of the implant, starting three threads from the implant head.
2.4
Statistical analyses
Statistical analyses were performed with Sigma Plot 13.x (SYSTAT, Chicago, Illinois). Qualitative data was graded as ordinal data and measurements of implant incorporation was conducted by grading the data starting with good = 2, followed by satisfactory = 1 and poor = 0. The presence of bone associated with the material was assessed as yes = 1 or no = 0. For the qualitative assessment, Mann Whitney Rank Sum test was used to compare the three different materials, and Wilcoxon Signed Rank test for comparison of the test materials with controls. For the histomorphometric data the Student’s t-test was used to compare the test materials and paired t -test for test material against control. The significance level was set at p < .05.
2
Materials and methods
2.1
Animals and study design
Eighteen adult male New Zeeland White rabbits from a specific-pathogen-free colony (Lidköpings Kaninfarm, Lidköping, Sweden) were used. The breeding colony was free from known rabbit pathogens according to recommended health monitoring [ ] At the time of surgery, the rabbits were 36 ± 4 weeks old and weighed 3.7 ± 0.2 kg (mean ± SD). The rabbits were housed individually in cages with a floor area of 0.42 m 2, equipped with a shelf and a covered area. Standard pelleted rabbit diet (Lactamin K3, Lantmännen, Stockholm, Sweden) and autoclaved hay were fed and rabbits had access to autoclaved straw for bedding and water ad lib . The light – dark cycle was 12:12 h with lights on at 07:00. Room temperature was 18 ± 3° C and humidity 55 + 10%. The animals were acclimatized for two weeks and accustomed to handling. On the day before surgery, the rabbits were clinically examined.
The study was designed as a prospective, randomised, controlled experiment and was approved by the Uppsala ethics committee for animal experiments (C 70/13). The animals were randomised in two groups (Microsoft Office Excel 2007, Microsoft, Kista, Sweden): Group CPC Granule (n = 9) and Group HABP·CaP Granule (n = 9). Furthermore, the side for placement of the test material was randomised to the maxillary sinus of one side and the control material, i.e. bovine mineral (Geistlich Bio-Oss ® , Geistlich Pharma AG, Wolhusen, Switzerland) was placed in contralateral sinus. The rabbits were also subjected to an unrelated study on the effect of the non-steroidal anti-inflammatory drug (NSAID) carprofen on bone formation [ ]. Within each material group, rabbits were randomised to receive either 5 mg/ kg of carprofen (Norocarp vet, 50 mg/ml, N-Vet, Uppsala, Sweden) or the equivalent volume of saline. The protocol ran for three months.
2.2
Material preparation
2.2.1
Calcium phosphate ceramic granules (CPC)
The preparation of the calcium phosphate compound was based on a previously published composition [ ]. The cement was prepared from mono-calcium phosphate monohydrate (MCPM), β-tricalcium phosphate (β-TCP), glycerol and deionized water. 0.4 mL of water and 4.5 g of glycerol were stirred into a homogenous liquid before it was added to a Falcon tube with a mixture of 11.2 g of MCPM and 13.8 g of β-TCP. The cement was first mixed in a cap vibrator (Ivoclar Vivadent AG, Schaan, Liechtenstein) followed by a quick mixing with a mortar and pestle. The cement paste was subsequently extruded through a 1 mm holed screen (Caleva bench-top screen extruder, Caleva process solutions Ltd. UK, Dorset) and shaped into granules (Spheronizer 120, Caleva process solutions Ltd. UK, Dorset).
The granules were sieved to obtain granules of a size between 0.60 mm and 1.18 mm, after which they were cured in 100% humidity at 37 °C for 24 h. The glycerol was removed by dissolution in a water bath for 48 h. The granules were then dried at room temperature for 24 h in a laminar flow cabinet. The final step was to autoclave the granules at 124 °C for 20 min. The phase composition of the granules was characterized by X-ray diffraction (XRD; D8 Advance, Bruker AXS GmbH, Karlsruhe, Germany). The granules were ground by mortar and pestle prior to testing. A structural characterization with a 2-theta setup was used with a beam knife, a Nickel filter and a Cu-K irradiation source. The resulting diffractogram was collected between 2θ of 5–60° at steps of 0.02° with 0.25 s per step and a rotation of 80 rpm. Phase composition of CPC granules from XRD analysis was β-CPP 6.1 wt%, β –TCP 10.4 wt%, Brushite 0.5 wt% and Monetite 82.8 wt%, followed by Rietveld refinement. The different crystalline phases were quantified by Rietveld refinement analysis, using BGMN software ( www.bgmn.de ) with Profex interface ( http://profex.doebelin.org ). The reference structures were PDF#04-008-8714 for β-TCP [ ] PDF#04-009-3876 for brushite [ ]. PDF#04-009-3755 for monetite [ ] and PDF#04-009-3876 for β-calcium pyrophosphate (β-CPP) [ ]. ( Fig. 1 ) All work was performed using sterile technique.
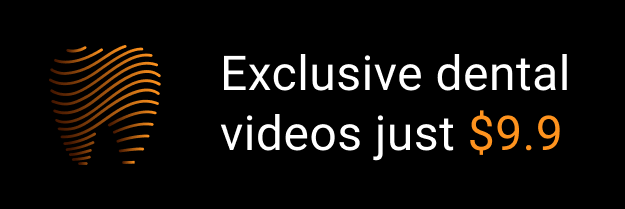