Abstract
Objective
Numerous bond strength tests have been performed on dental adhesion experiments. Yet, the validity of these bond strength tests is controversial due to the name ( e.g. , “shear” or “tensile”) may not reflect to the true and complete stress situation, i.e. , assumed uniform shear or uniaxial tensile conditions. Thus, the aim of this study was to simulate and compare the stress distribution of and between shear bond strength (SBS), tensile bond strength (TBS), mold-enclosed shear bond strength (ME-SBS) and de novo lever-induced mold-enclosed shear bond strength (LIME-SBS) tests.
Methods
3-Dimensional finite element method (FEM) was used on the dental resin-bonded surfaces ( i.e. , titanium alloy, dentine and porcelain) interphased with adhesive layer (thickness 5 μm) to simulate the mechanical tests. For ME-SBS, both polycarbonate and stainless steel molds were used. For LIME-SBS, stainless steel levers and molds with lengths of 3 mm, 6 mm, 12 mm, 15 mm and 18 mm were used. The applied loads on these models were 50 N, 100 N and 200 N.
Results
De novo LIME-SBS test was the most optimal configuration to evaluate “shear” bond strength of adhesive in regards to providing significantly high and uniform shear stress as well as eliminating tensile stress at the interface. The conventional SBS test created very high tensile stress at the load area, whereas the TBS created optimal tensile stress but shear stress indeed co-exist. The ME-SBS test could also eliminate some of the tensile stress. Similar stress distributions pattern appeared on the Ti-adhesive models, the dentine-adhesive models and porcelain-adhesive models.
Significance
None of the bond strength tests could give purely “shear” or “tensile” bond strength, but LIME-SBS seems to be the best model to evaluate the bond strength under true “shear” mode.
1
Introduction
Most dental materials operate in the hostile oral environment. In the past decades, much advancement of dental materials has improved their functionality in terms of strength, biocompatibility, aesthetics and so on. It is necessary to evaluate the functionality of these new materials in many aspects e.g. , bond strength. To assess the quality of dental adhesives performance on the bond, bond strength tests are necessary. According to ISO 1942 (Dental Vocabulary) , “bond strength test” is defined as “ mechanical test designed to measure the stress required to disrupt the bond between two materials ”. In clinical setting, the stresses for bonding may be comprised of various factors. It is inaccurate to assess overall bonding quality using any of the bond strength test, but at least predications could be made based on the bond strength test results.
Indeed, massive amounts of bond strength tests have been performed with regards to shear bond strength (SBS), tensile bond strength (TBS), micro shear bond strength (μSBS), micro tensile bond strength (μTBS), push out (PO), micro push out (μPO) and their several forms . Usually, macro tests are simple to carry out compared to micro tests. TBS tests are more uniform at the interface and are similar to nominal strength . In particular, shear bond strength test has been utilized for characterization of, e.g. , resin-to-resin , resin-to-metal , resin-to-ceramic , ceramic-to-ceramic , ceramic-to-metal , PMMA-to-metal , bondings. Prevalence of the shear bond strength test was high in laboratory testing, as the test does not require additional sample preparation compared to tensile bond strength test. Although “true” SBS is difficult to create and measure in conventional laboratory setting, this should possess as an important mechanical and physical property, i.e. , adhesive, of a material. For example, the push-out tests have been demonstrated as a method to test “true” shear bond strength .
In the conventional SBS test, usually a cylindrical adherent material is adhered to the adherend by adhesives ( Fig. 1 ). A tool ( e.g. , shear blade, chisel, metallic tape or wire loop) attached to the instrument crosshead could provide the load acting on the adherent among the test configuration ( Fig. 2 ) at shear . In many situations, the crosshead is usually applied at constant speed of 1.0 mm/min , although some other studies might also use other speeds, ranges from 0.5 mm/min to 5.0 mm/min . A load transducer connected with the crosshead would record the force. Once in contact with the sample, theoretically the crosshead counterforce would gradually rise, initiating from 0 N to a nominal value until fracture the sample. Then, the final counterforce at fracture is reported. The maximum recorded load ( F ) divided by the bonded area ( A ) is noted as one SBS value ( τ ) data for one unique shear bond strength test configuration ( τ = F / A ) . In fact, under the conventional shear condition, the test configuration formed by the adhesive, adherent and adherend might not always fully in ‘brittle shear’ mode. Indeed, the ‘ductile mode’ and ‘brittle-ductile mode’ are also observed by shear motion that would incur the cohesive or mixed failures.


It should be noted that the clinic performances and basic material properties should be two different parameters . The SBS should be considered as one mechanical property of the material. In contrast with the tensile bond strength test, the conventional SBS test has long been criticized for not be being appropriate or reliable to represent the so-called “actual” or “true” bond strength by shear at the bonding interface. The crack tip leading to the eventual fracture of the SBS test, could be caused by undesired high tensile stress, rather than the minimum required shear stress . The load is wasted in creating a cohesive failure within one substrate ( Fig. 2 ), rather than shear loading on the adhesive between the adherent and the adherend. Regarding the stress distribution, it has been revealed that the SBS test introduces stresses in shear that is non-uniform at the bonding interface as well as within the different substrates . That is, geometry of SBS test is inappropriate to measure shear bond strength . It has been also verified that the crack leading to eventual failure may have been resulted from a bending moment and high stress focused on the adherent ( e.g. , resin) . In this manner, a pre-mature failure is likely to occur. The same occurrence may also appear in μSBS tests . So, a SBS test may not be a test that is driven by shear stress only, but also caused by vast tensile stress. An optimal approach to test authentic bond strength at shear is still to be discovered.
In a TBS test, the sample is under tensile force until fracture occurred. Similar to SBS, the TBS is calculated as the force at fracture over the bonded cross sectional area. The stress distribution of a tensile bond strength test may also be localized. In the finite element analysis (FEA) of a tensile bond test, it was shown that the stress concentration at certain sites of the adhesion area also appeared . Yet, despite the TBS test is more appropriate in representing the bond strength in terms of tensile , there is no guarantee about TBS does not have any shear stress component. Furthermore, for laboratory preparation, the TBS requires the fine sectioning of a material which may lead to initial/eventual cracks in the sample, and might not easy to prepare specimen in, say, high strength materials.
Efforts have been made for restricting the undesired stress distribution in SBS test method. In some of the SBS tests, knife edge (chisel), stainless steel tape and orthodontic-looped wire were served as methods of loading . Using these methods, the length of the cylindrical adherent stub could be decreased since the point of loading could get very close to the adhesion surface. Apparently, shear stress would be more evenly distributed and partially diminished the tensile stress. Thus, this seems to be an commendable approach that could improve the SBS test . On the other hand, another rational approach, known as mold-enclosed shear bond strength (ME-SBS) test suggested by Van Meerbeek et al. , would be applying the mold to enclose the test stub, and the mold could provide a more evenly distributed load closest possible to the adhesion zone for the adherent. The ME-SBS test was suggested to eliminate the heterogeneous stress . This method is particularly useful and easy to operate since the retrieval of the mold before the SBS test is not required, and differences between independent measurements might be reduced. Cheetham et al. reported ME-SBS could exhibit a significantly higher mean μSBS and adhesive failure compared to non-enclosed specimens, and commented ME-SBS would give a more valid results when testing the bonding with metals . Meanwhile, the performance of ME-SBS in other adherends, e.g. , dentine and ceramics, are unknown, and the stress distribution in the test has never been studied and reported. Therefore, it might be useful to simulate the environment, such as using finite element analysis (FEA), in order to further understand the potential of the test, as well as to yield some theoretical background in the test development. FEA is a frequently used tool in engineering sciences to calculate the stress in materials. This technique virtually subdivides a volume into small elements. Then, the physical stress of each element could be computed with solving a set of equations simultaneously by FEA software. It has been deployed to analyze many dental materials under load . In similar studies, FEA was an ideal method to calculate the stress distribution along and within the interface of bonding site in shear and tensile bond tests .
To determine the “shear” component in bond strength test, the authors would like to suggest a novel lever-induced mold-enclosed shear bond strength (LIME-SBS) test ( Fig. 3 ). The concise concept requires modifications to the conventional SBS tests settings. A cylindrical adherent is bonded to block shaped adherend through adhesive, with enclosed mold. Then, a fulcrum is added into the shear bond strength configuration. The location of the fulcrum is set at the mid-point between the load and the adhesive interface. The load would be applied onto the mold at a relatively far distance from the adhesion zone. The stress distribution of the adhesive could be analyzed through FEA.

As a whole, the aim of this study was to exhibit and compare the stress distribution and magnitude of the adhesive layer in the SBS, TBS, ME-SBS and de novo LIME-SBS models with various substrates under FEA. The null hypotheses of this study are the SBS, ME-SBS and LIME-SBS tests do not differ in uniformity and magnitude of shear and tensile stress at the adhesion interface.
2
Materials and methods
The Abaqus software (version 6.14, ABAQUS Inc., Dassault Systemes) was used to create the 3-D models and analyze the stress at the bonding site. The bond strength test models were composed of 4 sections, i.e. , resin composite stub, mold (stainless steel/polycarbonate), adhesive layer and block material (titanium alloy, porcelain or dentine) ( Tables 1 and 2 ). The elastic modulus and Poisson’s ratio were given as input into software according to common values in literature as in Table 1 . All of the materials enlisted were set to be isotropic, homogeneous and linear-elastic. The mold is designed perpendicular to the block material. The interaction between mold and the block was defined as friction-free contact. The block was immobilized in all directions. An adhesive material at the bonding surface was created between the resin material and the block substrate. Its thickness was 5 μm . All degrees of freedom at the interface between mold and resin composite stub as well as between resin composite stub, adhesive and block material have been coupled to each other. The element type used in the mesh was hexahedral elements. For the analysis, the elements of resin composite stub, mold and block were approximately 0.15 mm. For meshing the 0.005 mm thick adhesive layer, the thickness was divided in two layers to prevent the model from serious distortion; therefore, the element size of each layer was set to 0.0025 mm in thickness.
Material | Young’s modulus (GPa) | Poisson’s ratio |
---|---|---|
Resin composite | 14.25 | 0.33 |
Adhesive | 1 | 0.3 |
Polycarbonate (PC) | 2.3 | 0.38 |
Stainless steel (SS) | 210 | 0.28 |
Dentine | 18 | 0.31 |
Ti alloy (Ti–6Al–4V) | 100 | 0.36 |
Porcelain (Vita InCeram ® Alumina core) | 271 | 0.19 |
Model | Mold | Resin | Load | Fulcrum | Adhesive | Adherend (titanium/dentin/porcelain) | |||||
---|---|---|---|---|---|---|---|---|---|---|---|
DØ inner (mm) | DØ outer (mm) | Height (mm) | Diameter (mm) | Height (mm) | Distance from adherend surface (mm) | Distance from adherend surface (mm) | Thickness (mm) | Length (mm) | Width (mm) | Thickness (mm) | |
SBS | No mold | 1.65 | 3 | 1 | / | 0.005 | 4 | 4 | 1 | ||
SBS(b) | No mold | 1.65 | 3 | 0.5 | / | 0.005 | 4 | 4 | 1 | ||
SBS PC mold | 1.65 | 3 | 3 | 1.65 | 3 | 1 | / | 0.005 | 4 | 4 | 1 |
SBS SS mold | 1.65 | 3 | 3 | 1.65 | 3 | 1 | / | 0.005 | 4 | 4 | 1 |
TBS SS | No mold | 1.65 | 3 | / | / | 0.005 | 4 | 4 | 1 | ||
L3 mm LIME-SBS SS mold | 1.65 | 3 | 4 | 1.65 | 4 | 3 | 1.5 | 0.005 | 4 | 4 | 1 |
L6 mm LIME-SBS SS mold | 1.65 | 3 | 7 | 1.65 | 7 | 6 | 3 | 0.005 | 4 | 4 | 1 |
L12 mm LIME-SBS SS mold | 1.65 | 3 | 13 | 1.65 | 13 | 12 | 6 | 0.005 | 4 | 4 | 1 |
L15 mm LIME-SBS SS mold | 1.65 | 3 | 16 | 1.65 | 16 | 15 | 7.5 | 0.005 | 4 | 4 | 1 |
L18 mm LIME-SBS SS mold | 1.65 | 3 | 19 | 1.65 | 19 | 18 | 9 | 0.005 | 4 | 4 | 1 |
The dimensions for all models are listed in Table 2 . In particular, the resin stub dimension in all models was all set in 1.65 mm diameter and 3 mm height. For all the models of SBS and ME-SBS tests, a rectangular indentation area of 0.2 mm × 1.0 mm was simulated for the blunt shear blade-shaped edge offering the load to simulate the shear blade edge as to mimic the practical situations. Moreover, it would avoid the stress concentration at the loading point and result in more accurate findings. This area was 1.0 mm away from the block material. To illustrate whether the well-known procedure of the load should be as close as possible to the block, another SBS model group, designated as SBS(b) group which was 0.5 mm away from the block material, was tested. For the LIME-SBS test models, the same indentation area was also created, with the distances to the adhesion site are listed in Table 2 . A fulcrum with rectangular area of 0.2 mm × 1.0 mm was added at the bottom of the mold in the mid-point between the load and the adhesion zone ( Table 2 , Fig. 3 c). It constrained the mold from vertical moving. So, this adjustment plays the role of fulcrum. All the molds in ME-SBS and LIME-SBS have outer-diameter of 3 mm.
In some previous laboratory studies, the maximum average strength for resin composite bonded to dentin with adhesives in SBS, μSBS, TBS and μTBS has been reported to be around 30 MPa, 60 MPa, 30 MPa and 80 MPa . Some individual samples may have even reached higher values. So, in this study, the applied load for each model was 50 N, 100 N and 200 N, which correspond to the final SBSs of 23.4 MPa, 46.8 MPa and 93.6 MPa. In the TBS test, the tensile load was applied on the entire free surface of the resin composite.
2
Materials and methods
The Abaqus software (version 6.14, ABAQUS Inc., Dassault Systemes) was used to create the 3-D models and analyze the stress at the bonding site. The bond strength test models were composed of 4 sections, i.e. , resin composite stub, mold (stainless steel/polycarbonate), adhesive layer and block material (titanium alloy, porcelain or dentine) ( Tables 1 and 2 ). The elastic modulus and Poisson’s ratio were given as input into software according to common values in literature as in Table 1 . All of the materials enlisted were set to be isotropic, homogeneous and linear-elastic. The mold is designed perpendicular to the block material. The interaction between mold and the block was defined as friction-free contact. The block was immobilized in all directions. An adhesive material at the bonding surface was created between the resin material and the block substrate. Its thickness was 5 μm . All degrees of freedom at the interface between mold and resin composite stub as well as between resin composite stub, adhesive and block material have been coupled to each other. The element type used in the mesh was hexahedral elements. For the analysis, the elements of resin composite stub, mold and block were approximately 0.15 mm. For meshing the 0.005 mm thick adhesive layer, the thickness was divided in two layers to prevent the model from serious distortion; therefore, the element size of each layer was set to 0.0025 mm in thickness.
Material | Young’s modulus (GPa) | Poisson’s ratio |
---|---|---|
Resin composite | 14.25 | 0.33 |
Adhesive | 1 | 0.3 |
Polycarbonate (PC) | 2.3 | 0.38 |
Stainless steel (SS) | 210 | 0.28 |
Dentine | 18 | 0.31 |
Ti alloy (Ti–6Al–4V) | 100 | 0.36 |
Porcelain (Vita InCeram ® Alumina core) | 271 | 0.19 |
Model | Mold | Resin | Load | Fulcrum | Adhesive | Adherend (titanium/dentin/porcelain) | |||||
---|---|---|---|---|---|---|---|---|---|---|---|
DØ inner (mm) | DØ outer (mm) | Height (mm) | Diameter (mm) | Height (mm) | Distance from adherend surface (mm) | Distance from adherend surface (mm) | Thickness (mm) | Length (mm) | Width (mm) | Thickness (mm) | |
SBS | No mold | 1.65 | 3 | 1 | / | 0.005 | 4 | 4 | 1 | ||
SBS(b) | No mold | 1.65 | 3 | 0.5 | / | 0.005 | 4 | 4 | 1 | ||
SBS PC mold | 1.65 | 3 | 3 | 1.65 | 3 | 1 | / | 0.005 | 4 | 4 | 1 |
SBS SS mold | 1.65 | 3 | 3 | 1.65 | 3 | 1 | / | 0.005 | 4 | 4 | 1 |
TBS SS | No mold | 1.65 | 3 | / | / | 0.005 | 4 | 4 | 1 | ||
L3 mm LIME-SBS SS mold | 1.65 | 3 | 4 | 1.65 | 4 | 3 | 1.5 | 0.005 | 4 | 4 | 1 |
L6 mm LIME-SBS SS mold | 1.65 | 3 | 7 | 1.65 | 7 | 6 | 3 | 0.005 | 4 | 4 | 1 |
L12 mm LIME-SBS SS mold | 1.65 | 3 | 13 | 1.65 | 13 | 12 | 6 | 0.005 | 4 | 4 | 1 |
L15 mm LIME-SBS SS mold | 1.65 | 3 | 16 | 1.65 | 16 | 15 | 7.5 | 0.005 | 4 | 4 | 1 |
L18 mm LIME-SBS SS mold | 1.65 | 3 | 19 | 1.65 | 19 | 18 | 9 | 0.005 | 4 | 4 | 1 |
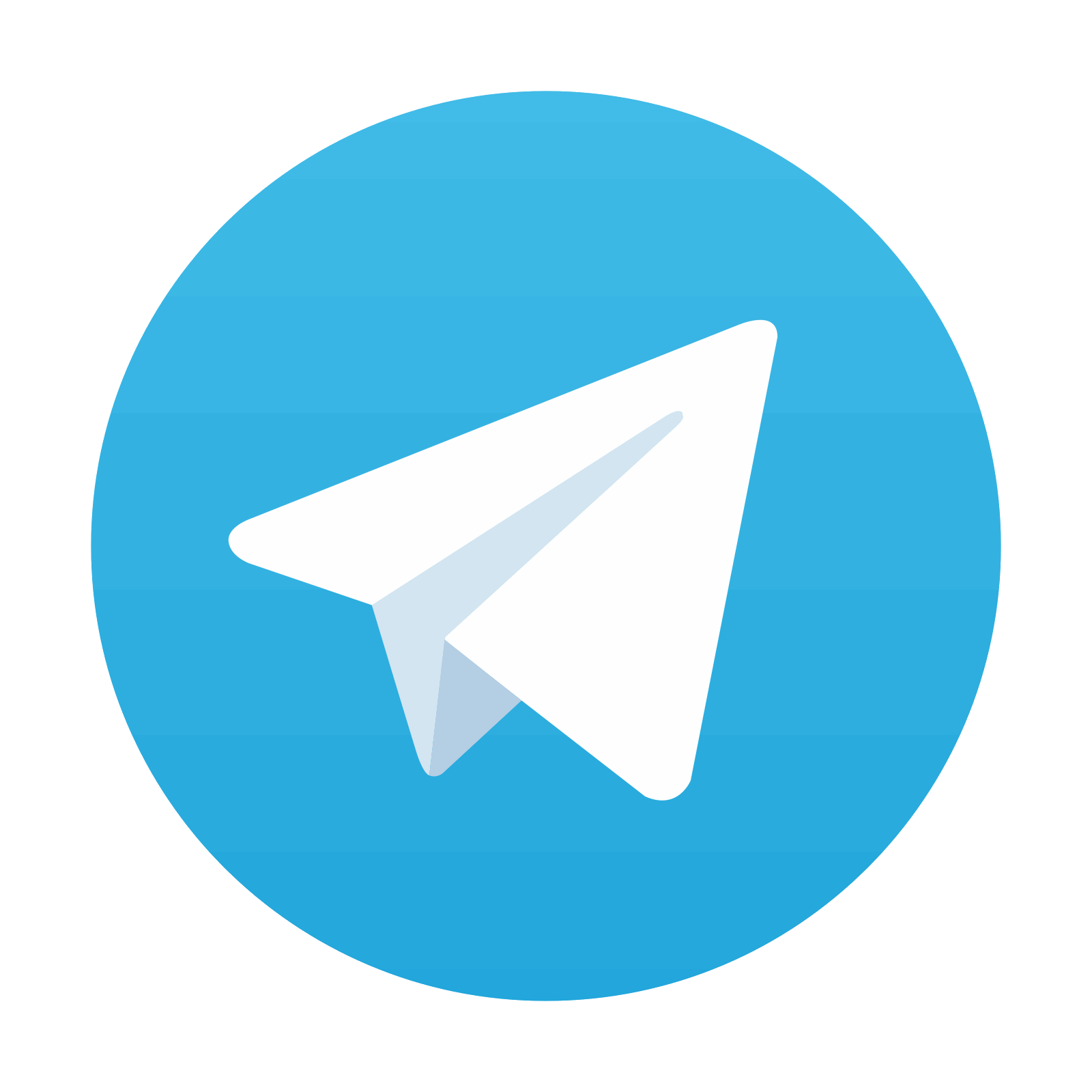
Stay updated, free dental videos. Join our Telegram channel

VIDEdental - Online dental courses
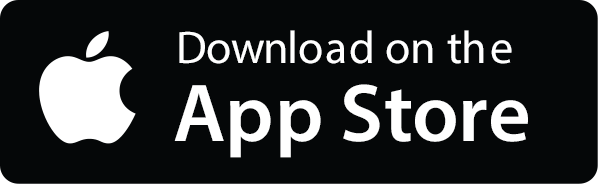
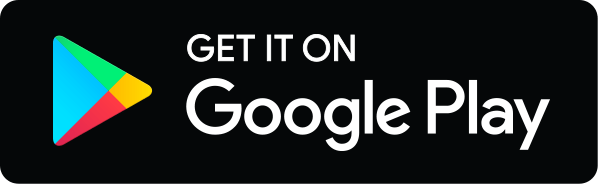
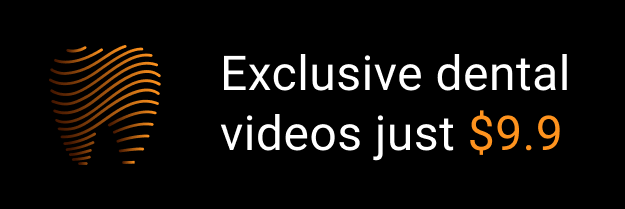