Abstract
This study explored the spatial variations in mechanical behavior of resin-infiltrated dentin using nanoscopic Dynamic Mechanical Analysis (DMA).
Objective
The objectives were to: (1) evaluate the mechanical behavior of resin-infiltrated dentin using a scanning-based approach to nanoindentation, (2) identify contributions of the collagen matrix to time-dependent deformation of the hybrid layer, and (3) assess the importance of specimen hydration on the nanoDMA response.
Methods
Specimens of completely demineralized dentin infiltrated with commercial resin adhesive and control samples of resin adhesive were evaluated using a nanoindenter in scanning mode. The load and displacement responses were used to perform DMA and to estimate the complex ( E *), storage ( E ′) and loss ( E ″) moduli over selected regions of evaluation. The importance of hydration on the mechanical behavior was also examined from a comparison of responses in the hydrated and dehydrated conditions.
Results
In the hydrated state the apparent complex, storage and loss moduli for the resin-infiltrated dentin samples were 3.5 ± 0.3 GPa, 3.4 ± 0.2 GPa and 0.9 ± 0.3 GPa, respectively. Those values for the resin adhesive control were 2.7 ± 0.3 GPa, 2.7 ± 0.3 GPa and 0.2 ± 0.02 GPa, respectively. Viscoelastic deformation of the resin-infiltrated collagen exceeded that occurring in regions of uniform resin adhesive. Though dehydration resulted in a significant increase in both the complex and storage moduli of the macro hybrid layer, the largest changes occurred to the resin adhesive.
Significance
The microstructure and hydration play critical roles on the mechanical behavior of the hybrid layer and nanoDMA provides a potent measurement tool for identifying the spatial variations.
1
Introduction
Resin composites have become the most common material for direct restorations, but there are concerns regarding their durability . Degradation of the hybrid layer over time has been identified as an important contributor . It is also possible that cyclic strain of the bonded interface induced by mastication causes accelerated degradation of the adhesive and hybrid layers . If this reduction in integrity facilitates loss of adhesion, then characterizing the microstructure and corresponding mechanical behavior of the hybrid layer is essential to understand the mechanisms of degradation.
Due to the physical scale of the hybrid layer and features of interest, transmission electron microscopy is often employed for evaluating its microstructure. However, the mechanical evaluations of this interface are generally performed by bond-strength testing using micro-tensile or micro-shear test methods. While the hybrid layer has been identified as the “weak-link” in composite restorations , the aforementioned test methods only indirectly describe properties of the interface. These techniques have limitations and the one most relevant here is that they cannot quantitatively describe the relationship between the microstructure and properties of the hybrid layer.
A structural evaluation of the hybrid layer requires a method with adequate spatial resolution. As the collagen fibrils, interfibrillar spaces and the remnant mineralized regions span from tens to hundreds of nanometers in scale, nanoindentation is an obvious candidate. Nanoindentation has found application in evaluating the properties of both hard (i.e. mineralized) tissues and soft tissues . Nanoindentation evaluations of dentin are not uncommon , and it has been adopted for examining both caries-affected and demineralized dentin as well . Collectively these studies reveal that sample hydration is important to mechanical behavior, especially in evaluations of dentin with low mineral content . One concern in the application of nanoindentation to dentin is the hierarchical organization of its constituents . The heterogeneous nature of the microstructure and scale of dominant features makes the indent size and placement critical to interpretation of properties . Single indents can provide erroneous information if placement is uncertain.
Evaluation of the hybrid layer using nanoindentation may involve regions of partially or fully demineralized dentin, resin-infiltrated dentin and resin adhesive. Single indents are incapable of adequately characterizing the distribution in properties of this region. A unique scanning approach to nanoindentation was recently applied for examining the effects of biomimetic remineralization on the mechanical behavior of dentin hybrid layers . In that study, nanoscopic Dynamic Mechanical Analysis (nanoDMA) was used to visualize and measure the elastic moduli of selected regions of resin-dentin bonds after water aging and biomimetic remineralization. In regards to dental materials, nanoDMA has been applied in both indentation and scanning modes for evaluating both intertubular and peritubular dentin . This approach has potential for characterizing spatial variations in properties of the hybrid layer, as well as the time-dependence in properties and the importance of structure on its mechanical behavior.
To the authors’ knowledge no study has systematically evaluated the use of nanoDMA and the influence of evaluation conditions for characterizing the apparent properties of the hybrid layer in dentin bonding. Thus, the objectives were to (1) evaluate the mechanical behavior of resin-infiltrated dentin using a scanning-based approach to nanoindentation, (2) identify contributions of the collagen matrix to time-dependent deformation of the hybrid layer, and (3) assess the importance of specimen hydration on the nanoDMA response.
2
Materials and methods
Hybrid layers created by contemporary etch-and-rinse adhesives are usually less than 10 μm thick. For this evaluation a larger sample was desired that was not subject to property gradients arising from variations in resin infiltration. Therefore, a “macro” resin-infiltrated dentin model was adopted involving a thick layer of demineralized dentin, which was originally developed for quantifying collagen matrix shrinkage during dentin bonding . Here, five macro-model specimens of resin-infiltrated dentin were prepared from demineralized dentin sections that were infiltrated with One-Step (Bisco Inc., Schaumburg, IL, USA), a contemporary dental adhesive. The dentin sections were derived from five extracted noncarious human third molars collected with informed consent under a protocol approved by the Georgia Health Sciences University Human Assurance Committee. A 2 mm thick disk of mid-coronal dentin was prepared perpendicular to the longitudinal axis of each tooth with a slow-speed Isomet saw (Buehler Ltd., Lake Bluff, IL, USA) under water cooling. The dentin sections were completely demineralized in 0.2 M formic acid/sodium formate (pH = 2.96) containing protease inhibitors to prevent collagen degradation, with the end point of demineralization monitored using digital radiography.
As the macro models of resin-infiltrated dentin were much thicker than the hybrid layers created during clinical bonding, a prolonged ethanol dehydration of water was employed to ensure optimal adhesive infiltration into the relatively thick demineralized collagen matrices . Infiltration of the adhesive was conducted under vacuum for 60 min under amber laboratory lighting to prevent premature curing of the light-curable dentin adhesives. After infiltration of the resin and curing the macro-hybrid layer samples were approximately 8 mm in diameter and approximately 2 mm thick. Each polymerized disk was mounted on a small steel disk with a very thin adhesive layer of One-Step, which was photocured for 40 s with a quartz halogen blue light emitting 600 mW/cm 2 . The steel mount was necessary to assist in the polishing process and to hold the sample on the magnetic base of the nanoindenter.
Polishing of the samples was necessary to remove excess resin and achieve a surface topography of the resin-infiltrated collagen matrix appropriate for evaluation by nanoindentation. It was recognized that a polishing process should be adopted that minimizes the potential formation of a smear layer. Thus, the samples were prepared following the methods reported in previous studies of dentin and polymers using nanoindentation and/or nanoDMA. Briefly, the macro-hybrid layer samples were polished using diamond particle suspensions (Buehler) of sizes 9 μm, 3 μm, and 0.04 μm with a standard cloth wheel. The polishing was performed by hand and without application of pressure. The surface roughness resulting from the method of preparation was quantified from the topography maps generated during nanoDMA scanning. In general, the surface roughness achieved using the aforementioned process ranged from between 10 and 40 nm. If an acquired scan exhibited an RMS roughness that exceeded 50 nm that scan was discarded and the location of evaluation was changed to a new area that exhibited a roughness lower than the defined limit.
Five One-Step resin adhesive “control” specimens were prepared from single drops of One-Step adhesive placed on a glass cover slip and then polymerized. The cover slip was placed directly on the steel mount for evaluation using nanoDMA. The surfaces of these samples did not require polishing as the surface roughness of the resin droplet was lower than 50 nm RMS as cured. The surface roughness of these samples was within the range of roughness of the polished resin-infiltrated dentin samples.
Scanning probe microscopy (SPM) of the macro-hybrid layer and control samples was performed using a Hysitron Triboindenter and a Berkovich diamond indenter with a 100 nm tip radius. In scanning mode evaluations, a selected surface area of the substrate is examined using a raster pattern with the indenter maintained under load or displacement control. Changes in the indenter height over the scan area quantify the topography, and the load and displacement signals are used to characterize the contact mechanics. In nanoDMA, a sinusoidal dynamic load is superposed onto the static contact load, which is akin to that used in conventional DMA ( Fig. 1 ). The dynamic components of load and displacement, along with their relative phase difference, are used in evaluating the dynamic elastic moduli including the complex ( E *), loss ( E ″) and storage ( E ′) moduli. Over 200 scans were performed to evaluate the apparent mechanical behavior of the five macro-hybrid layer and five control resin specimens utilizing nanoDMA. A scan window of 30 μm by 30 μm was utilized throughout. The contact load and displacement signals were acquired and used in estimating the E *, E ″ and E ′ of the specimens. Additional details regarding the underlying principles of nanoDMA can be found in the published literature .
The static contact load component used to initiate elastic contact (i.e. set-point load) was defined over a range from 1 μN to 4 μN, which was selected to achieve adequate contact with the specimen and to prevent permanent indentation of the macro-hybrid layer while scanning. A dynamic load of 50% of the static component ( Fig. 1 ) was applied in all evaluations, with a frequency of 100 Hz. In the nanoDMA study of Balooch et al. , a dynamic loading frequency of 200 Hz was used. In the present study both the dynamic/static load ratio and the loading frequency were chosen according to results of a parametric study aimed at identifying the influence of these parameters on the quality of the property maps and signal to noise ratio . These values also fall within the range of operating parameters recommended by the manufacturer. While the dynamic loading frequency is much greater than that of mastication, it provided the clearest property maps and strongest signal to noise ratio. The remaining measurement and analysis parameters, including scanning frequency (0.2 Hz), sensitivity (50 mV), and time constant (3 ms) were adjusted to optimize the scan characteristics and to acquire good spatial resolution in the moduli maps . The Triboindenter performs 256 horizontal scans over the vertical dimensions of the image space, which are then used in generating an image of the response with a resolution of 256 × 256 pixels. The time required to complete a scan is dependent on the scanning frequency, which denotes the frequency of horizontal scans (number per second). At 0.2 Hz frequency, the total scan time was just over 21 min (256 rows × 5 s per horizontal scan) for the 30 μm × 30 μm window.
NanoDMA scans of the specimens were performed in both dehydrated and hydrated conditions. It is important to note that the hybrid layer normally functions in a fully hydrated environment, thereby increasing the importance of evaluating the physical response in this condition. Hydration of the sample was achieved using a recently developed technique that enables scanning based nanoDMA . For all hydration testing, the specimens were submerged in water for at least 24 h to ensure complete hydration of the sample, i.e. particularly the surface region involved in the nanoindentation probing. The period of storage necessary to establish the state of hydration was identified by preliminary study in which further storage did not result in further changes in dynamic moduli. After removal from the hydrating bath, excess water was wiped from the surface and a drop of 99.4% ethylene glycol was applied to the polished sample. The drop was distributed evenly using a cloth saturated with ethylene glycol. Its use prevented the evaporation of water, while also eliminating problems related to the meniscus forces transferred from droplets of fluid to the indenter. The specimens were also examined after dehydration, which was achieved by free-convection in air for 24 h. Similar to achieving the state of hydration, the necessary period of storage was identified from preliminary study involving storage time and measurement of the dynamic moduli. After 24 h of dehydration there were no further changes in the moduli. Dehydration of the samples was performed after analyzing the samples in the hydrated condition. This order of analysis was chosen to prevent introducing artifacts in the samples by dehydration that would potentially be amplified by the dimensional changes occurring by rehydration. The E *, E ″ and E ′ of the macro-hybrid specimens obtained under dehydrated conditions and under hydrated conditions were compared using a one-way ANOVA with alpha set at 0.05.
Calibration samples of fused quartz and polycarbonate (one each) were obtained from the manufacturer and evaluated with and without ethylene glycol. The validation process was conducted to ensure that the method of maintaining the sample hydration did not cause adverse changes to the apparent mechanical behavior or introduce artifacts . Ten measurements were conducted on each sample, in each of the two conditions. Without ethylene glycol treatment, the average complex modulus ( Equartz=70.5±1.0GPa
E quartz = 70.5 ± 1.0 GPa
; EPC=2.9±0.1GPa
E PC = 2.9 ± 0.1 GPa
) obtained for both materials from nanoDMA was within 5% of the manufacturer’s reported reduced elastic modulus ( Er quartz = 69.6; Er PC = 3.1). The manufacturer did not evaluate the samples using nanoDMA so a direct validation of E *, E ″ and E ′ was not possible. The average complex modulus of the two materials obtained from nanoDMA with ethylene glycol treatment was Equartz=67.7±2.5GPa
E quartz = 67.7 ± 2.5 GPa
and EPC=3.1±0.1GPa
E PC = 3.1 ± 0.1 GPa
, which are also within 5% of the manufacturers values. According to a one-way ANOVA, there was no significant difference ( p < 0.05) in the moduli obtained for the fused silica with and without application of the ethylene glycol. It is important to the evaluation of the dynamic mechanical responses was conducted immediately after application of the ethylene glycol solution. There was a reduction in modulus of the polycarbonate sample after prolonged exposure, which was expected to occur due to plasticization of the polymer.
2
Materials and methods
Hybrid layers created by contemporary etch-and-rinse adhesives are usually less than 10 μm thick. For this evaluation a larger sample was desired that was not subject to property gradients arising from variations in resin infiltration. Therefore, a “macro” resin-infiltrated dentin model was adopted involving a thick layer of demineralized dentin, which was originally developed for quantifying collagen matrix shrinkage during dentin bonding . Here, five macro-model specimens of resin-infiltrated dentin were prepared from demineralized dentin sections that were infiltrated with One-Step (Bisco Inc., Schaumburg, IL, USA), a contemporary dental adhesive. The dentin sections were derived from five extracted noncarious human third molars collected with informed consent under a protocol approved by the Georgia Health Sciences University Human Assurance Committee. A 2 mm thick disk of mid-coronal dentin was prepared perpendicular to the longitudinal axis of each tooth with a slow-speed Isomet saw (Buehler Ltd., Lake Bluff, IL, USA) under water cooling. The dentin sections were completely demineralized in 0.2 M formic acid/sodium formate (pH = 2.96) containing protease inhibitors to prevent collagen degradation, with the end point of demineralization monitored using digital radiography.
As the macro models of resin-infiltrated dentin were much thicker than the hybrid layers created during clinical bonding, a prolonged ethanol dehydration of water was employed to ensure optimal adhesive infiltration into the relatively thick demineralized collagen matrices . Infiltration of the adhesive was conducted under vacuum for 60 min under amber laboratory lighting to prevent premature curing of the light-curable dentin adhesives. After infiltration of the resin and curing the macro-hybrid layer samples were approximately 8 mm in diameter and approximately 2 mm thick. Each polymerized disk was mounted on a small steel disk with a very thin adhesive layer of One-Step, which was photocured for 40 s with a quartz halogen blue light emitting 600 mW/cm 2 . The steel mount was necessary to assist in the polishing process and to hold the sample on the magnetic base of the nanoindenter.
Polishing of the samples was necessary to remove excess resin and achieve a surface topography of the resin-infiltrated collagen matrix appropriate for evaluation by nanoindentation. It was recognized that a polishing process should be adopted that minimizes the potential formation of a smear layer. Thus, the samples were prepared following the methods reported in previous studies of dentin and polymers using nanoindentation and/or nanoDMA. Briefly, the macro-hybrid layer samples were polished using diamond particle suspensions (Buehler) of sizes 9 μm, 3 μm, and 0.04 μm with a standard cloth wheel. The polishing was performed by hand and without application of pressure. The surface roughness resulting from the method of preparation was quantified from the topography maps generated during nanoDMA scanning. In general, the surface roughness achieved using the aforementioned process ranged from between 10 and 40 nm. If an acquired scan exhibited an RMS roughness that exceeded 50 nm that scan was discarded and the location of evaluation was changed to a new area that exhibited a roughness lower than the defined limit.
Five One-Step resin adhesive “control” specimens were prepared from single drops of One-Step adhesive placed on a glass cover slip and then polymerized. The cover slip was placed directly on the steel mount for evaluation using nanoDMA. The surfaces of these samples did not require polishing as the surface roughness of the resin droplet was lower than 50 nm RMS as cured. The surface roughness of these samples was within the range of roughness of the polished resin-infiltrated dentin samples.
Scanning probe microscopy (SPM) of the macro-hybrid layer and control samples was performed using a Hysitron Triboindenter and a Berkovich diamond indenter with a 100 nm tip radius. In scanning mode evaluations, a selected surface area of the substrate is examined using a raster pattern with the indenter maintained under load or displacement control. Changes in the indenter height over the scan area quantify the topography, and the load and displacement signals are used to characterize the contact mechanics. In nanoDMA, a sinusoidal dynamic load is superposed onto the static contact load, which is akin to that used in conventional DMA ( Fig. 1 ). The dynamic components of load and displacement, along with their relative phase difference, are used in evaluating the dynamic elastic moduli including the complex ( E *), loss ( E ″) and storage ( E ′) moduli. Over 200 scans were performed to evaluate the apparent mechanical behavior of the five macro-hybrid layer and five control resin specimens utilizing nanoDMA. A scan window of 30 μm by 30 μm was utilized throughout. The contact load and displacement signals were acquired and used in estimating the E *, E ″ and E ′ of the specimens. Additional details regarding the underlying principles of nanoDMA can be found in the published literature .
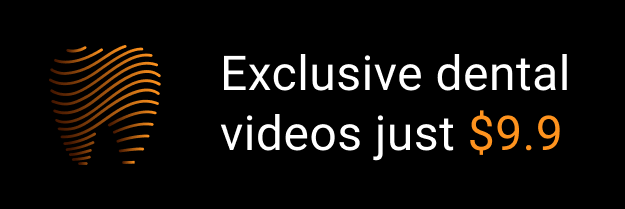