Cements and Liners
Cements in dentistry are composite materials based on mixtures of a solid and a liquid which, through an acid-base reaction, produce a solid matrix that binds the mass together. There are two types of task to which these materials are applied: to fill a hole in a tooth, that is, as a restorative material, and to retain an indirectly-fabricated device in place, such as an orthodontic bracket or a crown. In both cases the fixation may be either temporary or permanent, but it may also be fulfilled by other types of material such as filled resins (for both) and amalgam (as a restorative material). The appropriate choice depends on the mechanical properties, the appearance, the handling characteristics, and the behaviour in service. All of these depend entirely on the chemistry of the cement: composition, setting reactions, degradation mechanisms and structure. A detailed understanding of each is therefore necessary to make those decisions.
Zinc oxide-eugenol is an old, but still used material, although it has serious limitations. Zinc phosphate, too, has been in use for a long time. Its setting is dealt with in detail as a case study in the use of constitutional diagrams to understand the process. This stresses that the explanation of clinical observations and handling instructions can be obtained in no other way. Zinc polycarboxylate is also based on reaction with zinc oxide, but it offers the possibility of true adhesion as opposed to merely mechanical retention.
Ion-leachable glasses form a group of materials of increasing importance with the development of glass ionomer cements. These have the additional advantage of tooth-like appearance, but of this group only glass ionomer is adhesive.
An important additional property in the context of retention applications is film thickness, a purely geometrical consideration that affects the design of crowns and inlays. Cavity liners and varnishes are also discussed briefly.
The cements, as a group, illustrate very well the compromises that must be made in the design and use of dental materials. There are serious deficiencies in all types, and developments will no doubt continue. The benefits can only be judged by relating structures to behaviour.
Cements in dentistry have three general roles to play. The first is in the sense of a gap-filling mortar to join two solid objects together, such as an inlay or crown onto a tooth; in this context they are frequently referred to as luting agents. This sense is borrowed from the alchemists who were concerned to make air-tight seals in their apparatus by filling the gaps with a special paste or lute. Indeed, this idea of sealing is still relevant in dentistry. The second role, overlapping somewhat with the first, is that of an adhesive material for applying devices such as orthodontic brackets and buttons to tooth surfaces. The third job is of a direct restorative material, filling large spaces with a material which is intended to take masticatory loads and abrasion. The success or failure of a cement in any of these contexts depends on the chemistry of the setting process, on the structure of the resulting material, and therefore on the way that it is mixed and handled.
§1 General Principles
The basic principle behind all cements is that of a fluid, semi-fluid or plastic material which undergoes a change of state to a rigid solid and which therefore provides mechanical retention for one part on another. This change of condition may be achieved in several ways.[1]
•1.1 Change of state
The first is the simplest: the purely physical change of state involved in a material freezing, or at least being cooled to a glassy or highly viscous condition. Such a material is illustrated by sticky wax; the change is entirely reversible and no reaction with the substrate occurs. Retention of one part on another is due to the interlocking of the hardened wax with the roughness of both surfaces, and also due to the wetting of the substrate allowing van der Waals forces to operate (Chap. 10). But although sticky wax has great utility in the laboratory, this system or its like cannot be used effectively in the mouth because of the trade-off between two critical aspects.
Materials in general rapidly become weaker as their melting or softening points, Tm, are approached. The working or service temperature, T, must be sufficiently far below that temperature for the material to be useful, typically T/Tm < ~ 0.9 (temperatures in kelvin), assuming that the strength is high enough in the first place. Waxes are not strong (Chap. 16). Secondly, oral tissues cannot tolerate large temperature rises without severe damage; tissue temperatures of 45 °C are quite sufficient to cause severe inflammatory response, indeed, burns begin at around 44 °C. This combination of factors effectively prevents any material from being used in this context, as a suitable strength with a low enough melting point could not be obtained. For comparison, one could note that one application in dentistry where such a process is successful is soldering (Chap. 22); the temperatures here are much higher, and intrinsically stronger materials are involved – metals. However, even this is a second-class joint, not being as good as the metals being joined.
•1.2 Solvents
Solvent evaporation is the second means of effecting solidification. Materials relying on this process are usually called glues or adhesives. Solutions of polymers find application in a number of industrial and household products, the most obvious being water in gelatine (partially hydrolysed collagen) or starch glues, as used to be used for carpentry and bookbinding. Polystyrene and other ‘plastic’ so-called cements rely on the evaporation of volatile ketones, for example, while ‘rubber cements’ utilize petroleum fractions. Clearly the solvent must be matched to the solute for good solubility, and high concentrations of the solute are necessary to assist in gap filling.
However, there is no application in dentistry for the purposes of ‘cementing’ devices or restorations for this type of system. This arises from the need for there to be an appreciable rate of evaporation of the solvent for the strength of the cement to be high enough, early enough. There are time limitations in processes such as this in the clinic. Such evaporation cannot happen under impermeable metallic crowns or brackets, for example. Secondly, these materials are inherently very weak, relying for their effect on large surface areas of close contact, much as does sticky wax. Alternatively, when used on plastics, the solvent is meant to partially dissolve the substrate so that some chain mingling can occur, the most intimate ‘contact’ possible (cf. the repair of acrylic denture bases). But this means both a loss of surface precision and the application of a substance that will dissolve in the substrate polymer. Such solvents act as plasticizers, and therefore might permit the undesirable deformation of the object. They certainly cause swelling, and then shrinkage again when they finally escape (not necessarily in an accurately reversible manner). There is also the problem of toxicity. Many organic solvents are known to cause liver damage, and certainly must interfere with cell membranes (which are lipid) because they are solvents for fat-like substances. It is therefore inappropriate to expose the patient to them, or permit any to inhale the vapour unnecessarily. Thirdly, aqueous solvents are unusable in an aqueous environment as the cement would dissolve in the oral fluids. Even so, a major problem with these adhesives is the large shrinkage, or even porosity, which inevitably results from the evaporation of the solvent.
The only examples of these solvent-based systems in dentistry are found in the tray adhesives for impression materials, cavity varnishes and some dentine sealants, where strength is not a major factor. Some types of cavity liner essentially consist of calcium hydroxide or zinc oxide suspended in a volatile vehicle. These powders, having no appreciable cohesive strength on their own, may be bound together by a small amount of resin (i.e. a polymer) left behind when the solvent in which it was dissolved evaporates. Solvents such as ethanol or chloroform may be used in these circumstances. Generally, however, they are not satisfactory materials, failing to achieve what is intended with any reliability or longevity.
•1.3 Hydraulic reactions
Chemical reaction with water provides a third class of cementing materials, as typified by gypsum-based products and Portland cement. In these, water is a reactant. In general, such systems suffer problems of solubility in an aqueous environment, and rate of development of strength (very slow for Portland cement), while manipulative factors have generally excluded these and similar systems from dental use. Gypsum products, of course, are used in other contexts, but a variation on the theme of Portland cement is now available for specialized use in dentistry: MTA (§12). These types of system must be distinguished from those in which water is the medium for other reactions, whether or not it may be involved in a structural sense (cf. alginate and agar, Chap. 7).
•1.4 Acid-base reactions
Thus, because of the difficulties generally encountered, we find that in practice we are mostly concerned with one group: chemical reactions with compounds other than water, although it is true that water still plays a vital part in all dental systems. The chemistry of the class of materials represented by resins polymerized or cross-linked in situ by various means, which are often referred to as cements, have been dealt with elsewhere (6§4.7). While they may indeed have uses which overlap those of cements proper in the dental sense, the details will not be discussed further here. What we are left with, then, is a class of materials which are in fact based on the reaction between an acid and a base to produce some form of salt and, of course, water. These we may term the ‘true’ dental cements. Unfortunately, we are presently seeing a proliferation of products which try to combine resin-like chemistry with a cement-like system, combinations which confuse the issue. We shall return to these products later.
§2 Zinc Oxide – Eugenol
The oldest type of dental cement still in use is that produced by reacting zinc oxide and eugenol. Eugenol1 is the trivial name for a phenol (Fig. 2.1) whose special feature is the methoxy group at the 2-position, i.e. adjacent to the phenolic hydroxy group. Phenolic hydrogen is weakly acidic (Fig. 2.2) and may be removed to form a phenoxide ion. The strength of the acidity is about the same as that of the hydrogen in the bicarbonate ion, and so salts can be formed in an analogous manner:
< ?xml:namespace prefix = "mml" ns = "http://www.w3.org/1998/Math/MathML" />
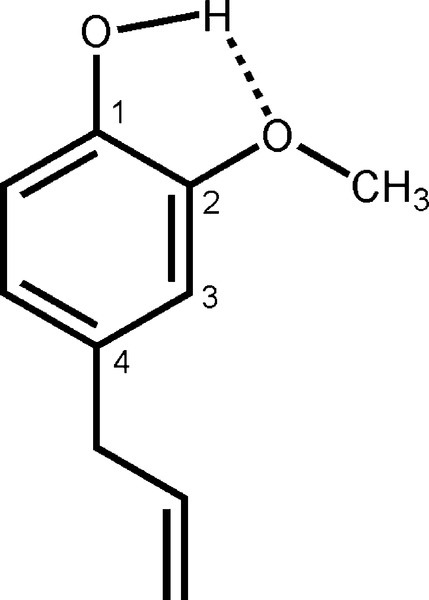
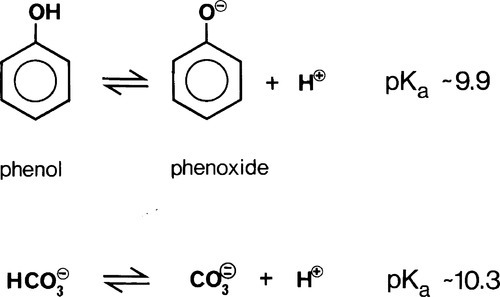
This is the setting reaction.[2]
Many multivalent metal ions can form complexes where they are coordinated to between 4 and 6 centres of high electron density. This coordination represents a state of often considerably lower energy than the dissociated ions and so may be very stable. Biological examples are the iron in haeme and the magnesium in chlorophyll. The environment of the metal ion is such as to shield as much as possible the strong positive charge it carries. This effect has already been seen for the calcium ions in alginates (7§9). Similarly, zinc ions react with the eugenolate ions formed by the acid-base reaction to form such a complex (Fig. 2.3). The molecule of water produced in the neutralization is also coordinated, lowering the energy even more. The essential point is the formation of the type of compound called a chelate (from the Greek word for a crab claw) by components which have the electrondense oxygen atoms in such a position as to form a ring readily. Five-membered rings, as formed here, are generally the most stable types of chelate.
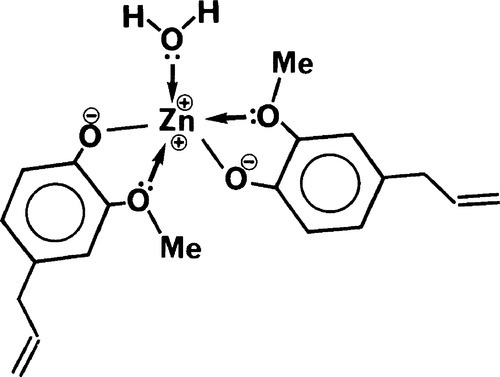
It is important to notice in that in this kind of cement the intermolecular bonds (i.e. between the complexes) are weak, being of the van der Waals type only. This sets a limit to the attainable strength of the material as a whole.
•2.1 Acceleration of setting
Depending as it does on the reaction of zinc ions with the eugenol, the setting process does not proceed at an appreciable rate in the complete absence of water – it is a solution-mediated process. Zinc oxide does dissolve slightly and slowly in water, but it then readily forms a tetrahedral hydrate which is unreactive. The hydrolysis of this to produce hydrated zinc ions is only slight, and proceeds too slowly for a clinically useful cement. Hence, any additive to the system to increase the availability of the zinc ions would be expected to improve the rate of setting. Quite clearly, water is going to be an accelerator as it permits the reaction in the first place. But the addition of hydrogen ions in the form of an acid would greatly increase the concentration of zinc ions by dissolving the oxide and hydrolysing any undissociated hydroxide. Zinc salts act in a similar way, both initially by raising the concentration of zinc ions, and subsequently because their consumption is accompanied by the liberation of hydrogen ions. Thus the pH will drop and the dissolution of zinc oxide increases. Commercial products take advantage of these effects in their formulations, small additions of water and zinc acetate or acetic acid being made. Even so, the setting rate is markedly affected by the ambient humidity at the point of mixing, and care must be taken in the storage and use of such materials to avoid excessive variation (Fig. 2.4).[3] On the other hand, water present at the surface of dentine will encourage setting and there is no point in attempting to dry this other than to remove evident liquid.
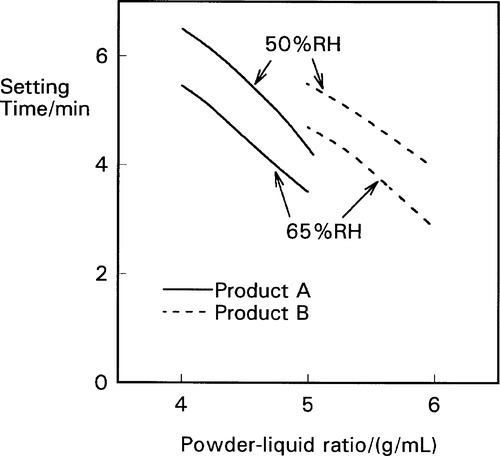
•2.2 Dissociation
Unfortunately, the chelate formed with eugenol is not particularly stable and the reaction is slowly reversed in the presence of excess water:
Thus this is, in fact, an equilibrium, lying to the left here because of the excess zinc oxide. However, since eugenol is slightly soluble in water and volatile, it will thus diffuse away from the site and escape from the system. This steady hydrolysis limits the life and applicability of the material as a cement. Indeed, even as a liner – where one might imagine it to be isolated from its surroundings – it may not be very long lived. There are reports that it has disappeared from beneath amalgam restorations, presumably dissolved in the fluid present, which is percolating because of a lack of seal (14§6.2).
Furthermore, it is not a particularly strong or abrasion resistant cement. Attempts have been made to improve the material by using various additives such as resins which may dissolve in the eugenol and provide some further binding when set as well as modifying the rheological characteristics when freshly mixed. None has achieved any prominence.
Zinc oxide-eugenol cement (or ‘ZoE’) has been frequently described as obtundent (“dulling or blunting sensibility”), or even “palliative”, yet there seems to be no evidence of any beneficial effects under such material: there is no reparative dentine, and only inflammatory responses are found, mostly ascribed to the eugenol. It would appear that the pulp is, at best, damaged by these materials. In addition, eugenol is a known sensitizer, and may provoke a strong physiological response in some persons.
•2.3 Discolouration
The presence of the phenolic hydrogen in eugenol and similar substances has an untoward effect on resin restorative materials placed on top: it inhibits polymerization because it is a free radical sink (5§4). It will thus prevent proper curing at the bottom of the restoration. Furthermore, eugenol and other substances that may be included in the cement’s liquid will dissolve in the restorative resin, discolouring it in the process to a rather dark yellow. This will not occur immediately but over some hours, effectively destroying any colour match that may have been achieved. There will of course be the other consequences of exposure to a plasticizer: softening and swelling – and therefore distortion, then eventual shrinkage.
Eugenol is itself subject to attack by oxygen, and suffers reactions akin to those shown in 6§6 (cf. Fig. 5§4.2) probably aided by exposure to UV light. These reactions are responsible for the gradual darkening of the liquid in storage, despite the use of brown glass bottles. As will be noticed, eugenol has a vinyl group which would be expected to be polymerizable, and although the competition with ‘inhibitory’ aspects of its chemistry complicate matters, there is some evidence that such a reaction may occur slowly in ZoE to yield a complex polymer network very much like lignin (the principal binding polymer of wood).[4] Indeed, lignin is formed from coniferyl alcohol and similar molecules, to which eugenol is closely related. While it is not clear whether discoloured eugenol is appreciably affected in terms of its reactivity with zinc oxide, if the polymerization reaction could be promoted it might lead to a cement which is both stronger and less soluble, even though this would not affect the initial irritancy or the effect on resins.
•2.4 Variations
In view of the general weakness of zinc-oxide-eugenol cement, many attempts have been made to improve on it. These attempts include the use of inert fillers, polymeric binders dissolved in the eugenol (as mentioned above), and a wide variety of other formulations. In addition, alternative chelating agents have also been much investigated, for there are many compounds meeting the basic structural requirements for chelation (Fig. 2.5) and indeed these have given rise to various commercial products. In each case, a more or less acidic hydrogen can be replaced by the metal ion.
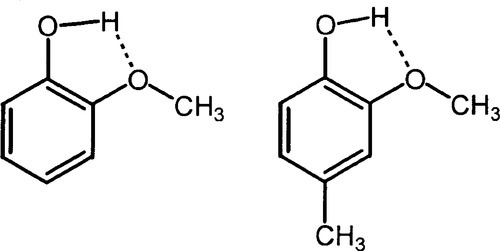
The most successful of these alternatives seems to be 2-ethoxybenzoic acid or ‘EBA’ (Fig. 2.6). Being a carboxylic acid, this is much more acidic than eugenol, and therefore much more reactive in salt formation than the phenol; this also leads to fewer problems with control of the setting rate. However, the more strongly polar benzoate is more readily solvated by water and the solubility of the set cement accordingly much greater. The six-membered ring is perhaps a little less stable, although probably offset by the increased polarity of the carboxylate. Even so, with the addition of inert filler, a proportion of eugenol and other materials in small quantities, some slight net improvement of properties over plain zinc oxide-eugenol can be obtained. The principal advantage, however, seems to be a reduced tendency to cause a burning sensation as does eugenol on its own.
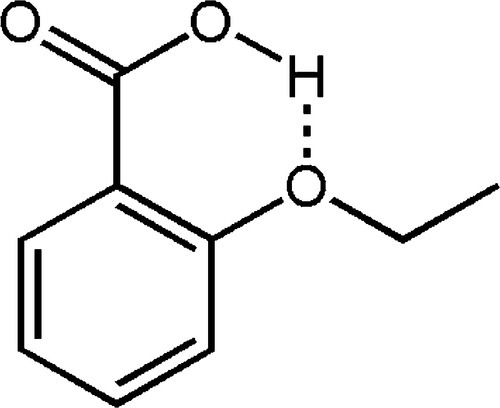
•2.5 Presentation
Originally sold just as powder and liquid, ZoE cement has also been presented as a two-paste system. Here, the zinc oxide and accelerator will be suspended in an inert vehicle, an oily or resinous mixture that plays no part in any reaction. In addition, the eugenol is made into a paste with an inert filler. The main advantage of this approach perhaps being apparent ease of dispensing and mixing. Certainly, no great improvements in mechanical properties are expected: the inert filler is unbonded and the vehicle for the ZnO can only weaken the system. The use of the label “catalyst” for the eugenol component is also incorrect for these materials (cf. 7§4.2), and “accelerator” is similarly unhelpfully wrong.
Zinc oxide-eugenol is also used as an impression material in prosthetic dentistry, when it is called a ‘paste’ rather than cement, indeed, it is often presented in the two-paste form. There is no fundamental difference between this and the cement: the setting reaction is the same. Some adjustments may be made to the proportions of the reactants and inert components to adjust the rheological properties of the unset material, and the pastes may be coloured to assist in monitoring their mixing (15§7).
§3 Background Chemistry
•3.1 Zinc oxide
Zinc oxide is used in a remarkable number of dental products, mostly cements,[5] for reasons of reactivity, radio-opacity, whiteness, and low cost, but also – given those other benefits – low toxicity. Zinc is an essential element in human physiology as a component of many enzymes, but is apparently tolerated at levels well above those needed to maintain health. This may account for the widespread use of zinc oxide and the absence of overt problems in biological contexts. A little background chemistry is helpful in understanding some of behaviour in certain applications.
Zinc oxide exists in the so-called wurtzite crystal structure (Fig. 3.1) which may be viewed as a network of tetrahedra formed by the oxygen atoms in a structure reminiscent of that of diamond.[6] It can be seen that of the possible tetrahedrally-coordinated sites in the structure, between groups of oxide ions, only half should be occupied by zinc ions in order to satisfy exactly the stoichiometry of the composition ZnO. If zinc oxide is heated, a slight decomposition occurs:
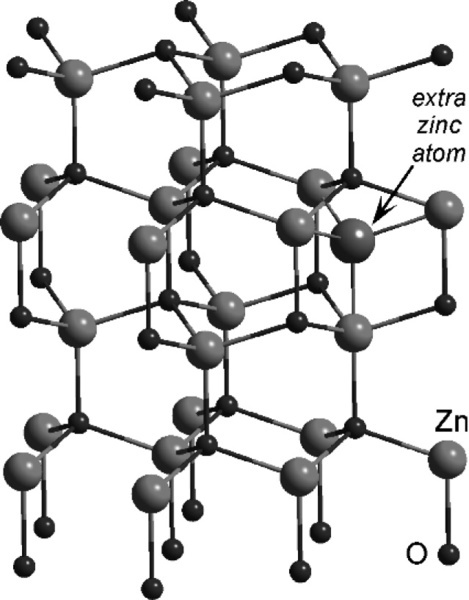
which effectively removes some oxygen atoms from the lattice. The excess zinc atoms (not ions, notice) then dissolve in the remaining solid oxide and occupy some of the vacant holes (i.e. interstitial sites). The extent of this depends on temperature, and up to 0.03 atom percent of Zn in excess of the stoichiometric ratio may be present. This results in a slightly coloured material, ranging from pale yellow to deeper shades. As can be deduced from the equilibrium above, Zn vapour will increase the colour and oxygen gas will bleach it.
The important point in the present context is that the presence of the excess Zn atoms reduces the reactivity of the oxide with respect to acid-base reactions. In the case of the reaction with eugenol this leads to excessively long setting times, especially as the reaction is sluggish to start with, and care has to be taken with the preparation of the oxide for this reason. Reactive zinc oxide can be made by decomposing by heat the carbonate or the hydroxide at low temperatures. On the other hand, heating to high temperatures can be used deliberately to reduce reactivity. For these reasons, zinc oxide supplied with one type of product should not be used with any other product, or even a different brand of the same type of product. The reactivity is likely to be wrong.
•3.2 Oxide selection
The choice of zinc oxide for so many uses may seem a little odd, until one arrives at its near-inevitability by a process of elimination. Taking out from the list of elements those that are firstly chemically-inappropriate as not being metals, those that are extremely reactive, radioactive or otherwise grossly toxic, then those that are strongly coloured, finally those that are simply too expensive (bearing in mind that these are frequently overlapping categories, not mutually-exclusive labels), we are left with eight possibilities (Fig. 3.2). Of these, Mg, Al, Ca and Ti are not very radio-opaque with respect to tooth tissue (Chap. 27), and Sn can be toxic, simply because it is a heavy metal – as are Sr and Ba, when in a soluble form. That leaves Zn. This is not to say that in the right context, with strong enough justification or special chemical context, others might not be used (cf. §10), on balance it turns out to be, at the least, a convenient material.
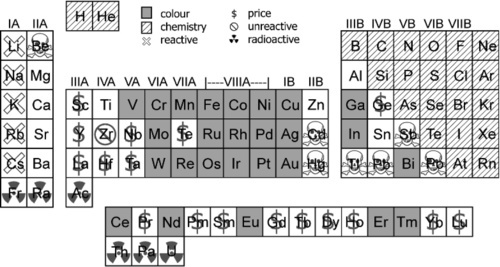
•3.3 Acidity
Reference is often made to the relative weakness or strength of an acid (or base) (e.g. §2, 7§9.1). It is well to remember that this phrasing refers to the ease or completeness of ionization:
Given that this dissociation is an equilibrium, we write:
where Ka, the equilibrium constant for reaction 3.2, is specifically labelled the acidity constant. Since the range of possible values is very large, and the values themselves typically rather small, it is simpler to use the negative logarithm:
– in the manner of the definition of pH. It should be noted that this is measure of how easily the anion is made available for reaction, and the strength of the anion as a ligand in some contexts (although several other factors are involved). Thus, talking about the strength or weakness of an acid does not refer to concentration, despite the fact that in common speech a ‘weak’ solution, for example, means a dilute one. Again, care with terminology is required.
Furthermore, acidity as such is not a direct measure of the rate at which reactions will occur, as this depends on many kinetic factors as well as concentration, and does not necessarily indicate relative corrosiveness towards metals, for example.
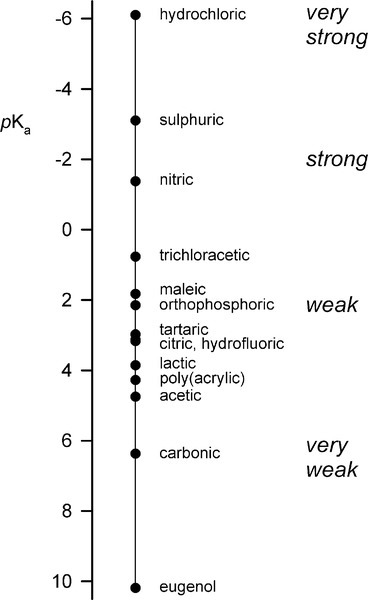
§4 Cements as Composite Materials
It is most important to realize that with the zinc oxide-eugenol system, as indeed with all other dental cement systems discussed here, the set material is essentially a composite structure. Always much more of the solid component (and not just zinc oxide) is used than is required for complete reaction with the liquid, and so it persists as an appreciable volume fraction of the set material as a stiff and relatively strong core in the matrix of reaction products. Hence, as was found for the filled-resin restorative materials, the higher the powder : liquid ratio, the higher both the modulus of elasticity and the strength of the set cement are expected to be.
Naturally, there is a limit to the amount of powder that can be used because the viscosity of the mixture rises rapidly (4§9) and would easily become unworkable at high ratios. This is apart from the more critical limit set by the minimum mixing volume of liquid required (2§2). Porosity will rise rapidly if this requirement is not met, with the usual effect on strength (Fig. 2§8.3). It must be remembered, however, that in general mixing powders with viscous liquids to make thick pastes is likely of itself to include air bubbles regardless of the presence of the ‘correct’ amount of liquid. As a result all set cements are inevitably porous to a greater or lesser extent and thus have their strength limited according to the Griffith criterion (1§7). Mixing and handling techniques must endeavour to minimize this intrinsic defect and therefore its detrimental effects on the reliability, longevity and efficacy of the treatment of which it is a part.
It is worth emphasizing here, however, that the minimum mixing volume for cements is required to be less than the stoichiometric amount for complete reaction if there is to be a core remaining. This is in contrast to the situation for gypsum products and investments, where the minimum mixing volume is required to be at least that necessary for complete reaction. Given that such mixtures are plastic dilatant (4§7.7), it would be expected that at the completion of the procedure the surface must still be glossy if the requirements have been met. This provides a simple visual check of the adequacy of the mixing ratio.
It must be assumed that there will be changes in total volume between reactants and reaction products, and since in general there is a reduction in the mobility of the components this change must be a net shrinkage (by a reduction of free volume), although contact between core and solid reaction products means that a loss-of-gloss event can be anticipated. In addition, crystal growth pressure effects may be present when crystallization is involved. However, since the scale of use is small, the absolute value of the dimensional change is probably insignificant in most contexts.
Porosity not only affects strength, it affects modulus of elasticity as well, and this applies to all classes of materials, not just cements: impression materials, acrylic, amalgam, investments, porcelain, and so on. As might be deduced from a consideration of the rules of mixtures (6§2), the behaviour is complicated. It can be approximated for closed porosity, which pores are assumed to have Ep = 0 and volume fraction ϕp, by
where A is a function of the Poisson ratio, ν (Fig. 4.1).[7] It is therefore clear that a porous body must be treated as a composite with the porosity as a phase. Thus, wherever the true value of a non-porous material elastic modulus is of interest and this is to be determined with a macroscopic experiment, any porosity present must be taken into account, and vice versa. In other words, where stresses or strains are to be found in an experimental or service context, the effect of the porosity must be recognized, quite apart from any flaw-related strength considerations, as it clear that there is considerable sensitivity.
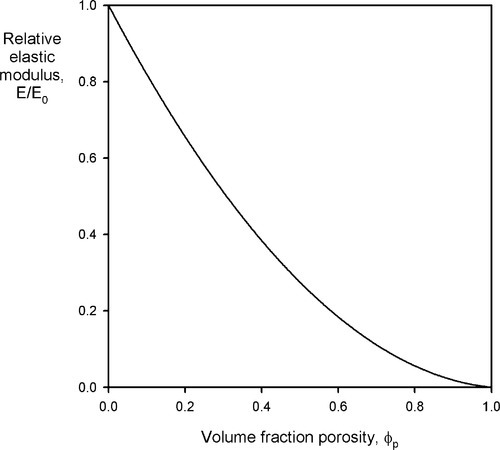
Machine mixing of encapsulated cement products may result in higher porosity,[8] with air being ‘beaten into’ the mixture, and this may even be exacerbated by the lower powder : liquid ratios used in order that this kind of mixing procedure works at all (15§3). The lower ratio must mean lower strength anyway, but the increased porosity would make it worse still.
§5 Zinc Phosphate
Another cement system based on zinc oxide that has been used in dentistry for a very long time is that which relies on the reaction with orthophosphoric acid.2 The overall reaction resulting from simply mixing zinc oxide and aqueous phosphoric acid is essentially:
This, however, is ordinarily a very fast reaction, and since it is highly exothermic (H+ + OH– releases 57 kJ/mol) the temperature rises greatly. When the mixture cools this results in a friable mass of crystals which is of no use as a cement, even were it possible to get it into place before setting. The problems are therefore threefold:
• to reduce the rate of setting,
• dissipate the heat (the total amount of which is, of course, entirely unaffected by the rate of setting), and
• improve the strength in order to obtain a practical cement.
•5.1 Oxide modification
As mentioned above (§3), the reactivity of the zinc oxide can be altered by the method of preparation, and for phosphate cements this means firing at a very high temperature for some time. This may have several effects. Firstly, the excess Zn0 atoms (§3) affect reactivity. Secondly, sintering will occur to reduce surface area, which will involve both particle roughness and agglomeration, as well as remove some kinds of crystallinity defect, again reducing reactivity. Magnesium oxide is added as this also reduces the reactivity, and indeed other oxides such as those of aluminium and silicon may be added for the same reason. In addition, the presence of the aluminium may suppress crystallization of the reaction product (§5.15), and other precipitation may occur to coat the oxide particles with a diffusion barrier (cf. 2§7.4). Unfortunately, little seems to be known for certain in this area, the products as sold are empirically derived – i.e. from trial and error.
•5.2 Liquid
The liquid might present another possibility for controlling reaction rate but this is, in fact, insignificant in practice. Orthophosphoric acid is a relatively weak acid (although much stronger than eugenol), and the ions present in solutions containing it depend on the pH, such that at pH 0 only very small amounts of may be present (Fig. 5.1). Similarly, because pH falls steadily with increasing concentration through the range of dental interest (Fig. 5.2), the only ionic phosphate species effectively present is
and then in low concentration. It is only at very low overall phosphate concentrations or much higher pH that other ions appear. Therefore the ionic constitution of the acid liquid is essentially irrelevant to the setting reaction of zinc phosphate cement3 and its rate. This is contrary to the statements sometimes made that the reaction products depend directly on the proportions of the ions present, a misunderstanding of the nature of equilibria.
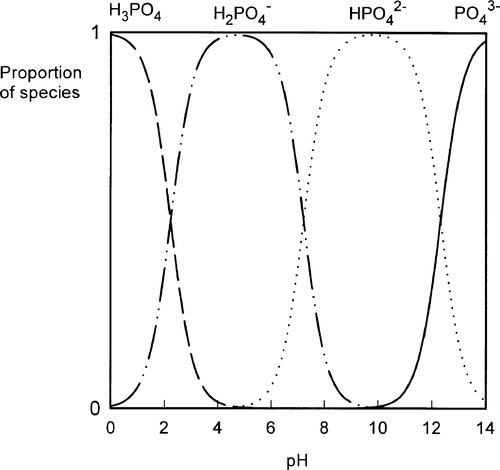
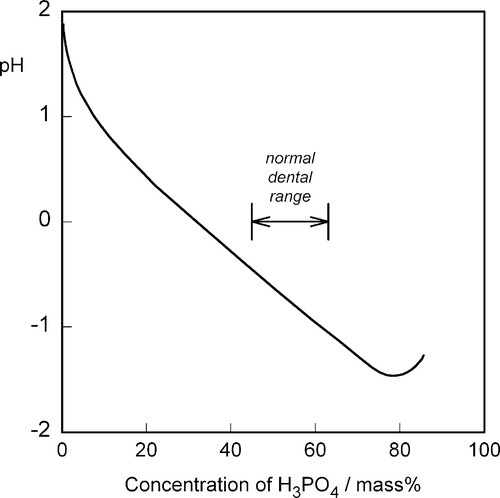
The chemistry can nevertheless be understood by examination of the P2O5 − H2O − ZnO constitutional diagram. This turns out to show the power of such an approach in dealing with a system as complicated as this one, where elementary acid-base considerations fail completely to explain both the empirically-derived clinical handling procedure and known properties.[9] In that sense, this system may be treated as a case study to put into a practical context a number of the points made in Chap. 8, and for that reason the explanation will be detailed and stepwise through the entire process.
•5.3 Diagram layout
To study the chemistry of this system, we start by identifying the known stoichiometric compounds and plotting these on a ternary composition diagram (Fig. 5.3). Since we are only dealing with whole-number ratios of the components (even though the empirical formulae may be reduced to the lowest numerical terms by taking out common factors), various isopleths can be drawn through those compound points to indicate the relationships: e.g., the sequence from zinc metaphosphate, Zn(PO3)2, towards the water apex, adding two, then two more, moles of water to form the primary phosphate, then its dihydrate. Similarly, a line drawn between any of the water-free salt points (on the ZnO − P2O5 edge) and the H2O apex are lines of constant Zn-P ratio, and the corresponding hydrates therefore lie on such lines. This kind of plot is a purely numerical exercise: there is no necessarily implied chemistry. However, the line between the points corresponding to the compositions of H3PO4 and Zn3(PO4)2 does, for example, represent the anhydrous reaction products of the neutralization of orthophosphoric acid itself by ZnO, including the compound Zn(H2PO4)2 ⋅ 2H3PO4, which has acid of crystallization.
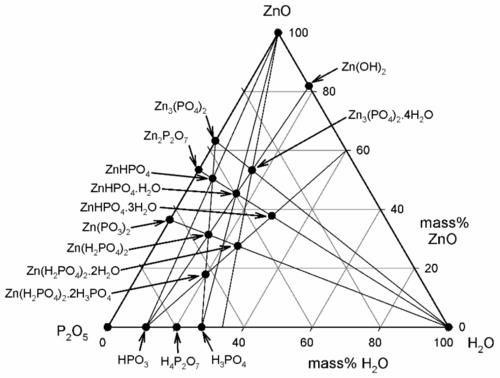
One matter of immediate interest is the orthophosphoric acid point, H3PO4. The edge of the composition triangle between this and the water apex can be recalibrated as the concentration (mass%) of this acid in aqueous solution (Fig. 5.4). This is an example of the application of the lever rule (8§3.10). The formation of H3PO4 can be written thus:
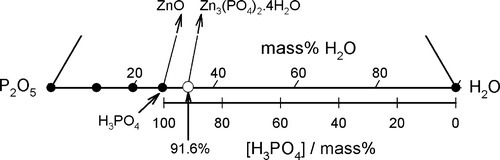
It can then be seen that the isopleth which runs through the point for Zn3(PO4)2 ⋅ 4H2O identifies the acid concentration required for complete reaction, as in equation 5.1.
The isothermal section of a partial equilibrium constitutional diagram at 25 °C is shown in Fig. 5.5, with several special points labelled. The detail of the area of the diagram on the left-hand side is omitted as it is of no relevance to the dental system and can safely be ignored. In addition, zinc hydroxide has not been reported to form under normal dental conditions, so can be omitted from consideration. As indicated above, the effective mixing liquid of the dental product is H3PO4 solution, so that composition line is used for clarity, rather than P2O5 − H2O. To simplify the description, phase labels as in Table 5.1 will be used.
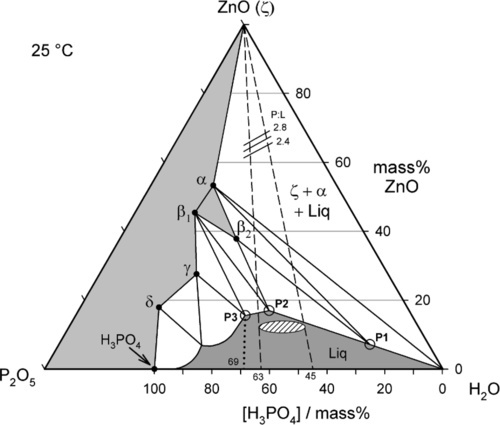
Table 5.1
Phase labels for zinc phosphates relevant to the dental cement system
α | Zn3(PO4)2 · 4H2O |
β1 | ZnHPO4 · H2O |
β2 | ZnHPO4 · 3H2O |
γ | Zn(H2PO4)2 · 2H2O |
δ | Zn(H2PO4)2 · H3PO4 |
The compounds of relevance are marked by the solid points with the phase labels. The solidus, the boundary between the regions of all solid and those of solid + liquid, is represented by the irregular line drawn between the points ZnO – α – β2 – β1 – γ – δ – H3PO4. The liquidus, the boundary between the regions of all liquid and those of solid + liquid, is the line drawn between the points H2O − P1 – P2 – P3 – … – H3PO4. The points P1 – P3 do not correspond to stoichiometric compounds, but are critical solution compositions at 25 °C. These will be explained below.
The extremes of the reported commercial range of orthophosphoric acid concentrations for the dental cement, approximately 45 ~ 63 mass% as H3PO4, are marked on the acid composition line (Fig. 5.5). Since the dental cement is prepared by mixing zinc oxide with just one such acid liquid, isopleths can be drawn from the acid line to the ZnO apex (constant P2O5 : H2O ratio = constant H3PO4 : H2O ratio) to represent all such possible mixtures. The dashed lines then represent the limits to the overall compositions which may be obtained with those commercial liquids, that is, at all possible powder : liquid ratios.
The reported upper limit of about 10 mass% Zn (i.e. calculated as metal) in those same commercial liquids corresponds to the region of the hatched ellipse in the liquid field in Fig. 5.5. It will be seen that the incorporation of zinc in the liquid represents an approach to the liquidus, with the consequent benefit of partial neutralization of the acid and therefore the loss of that portion of the heat of reaction prior to clinical use. In addition, the reduction of the concentration of ‘free’ acid means that its reactivity is also reduced, so the initial rate of evolution of heat is also lower. The more zinc or other metal dissolved in the liquid, the closer to the liquidus does its composition lie, and the more sensitive to loss of water it will become, when solid might appear. Cloudy liquids, or ones containing crystals, are spoilt and should be discarded, as should the remains of liquid from a pack whose powder has been used up. (This, of course, applies to any powder-liquid cement system, not just zinc phosphate.)
Commonly, the liquid is said to be ‘buffered’ by the zinc, and sometimes other metals such as aluminium that are present in the liquid as supplied. Given the remarks above about the constitution of the liquid, and the reduction in the total heat of neutralization that this dissolved metal represents, such a description is seen to be of little value and plays no part in understanding the system.
•5.4 Setting reactions – Stage 1
To consider the setting reactions of the dental cement, we conduct a thought experiment in the form of imagining the consequences of a very slow addition of ZnO to the chosen acid composition, performing a titration of the acid with a base. This is realistic in the sense that it is the ZnO that dissolves in the acid, and not the other way around. Thus, if we take the liquid composition as, say, 45 mass% H3PO4, the first step is to draw the mixing line from that point on the acid composition line to the ZnO apex (Fig. 5.5). This is then a composition line itself, representing all possible mixtures of the acid liquid and the powder. We can also refer to Ostwald’s Rule of Stages (8§2.6) to describe the sequential formation of successively more stable phases, noting that α ‐ Zn3(PO4)2 ⋅ 4H2O is not stable in contact with the original liquid.
It is worth stressing that such a mixing line represents the only (overall) compositions accessible when mixing a given liquid with ZnO – it is only the proportions of these two that can change. Hence, in doing the thought experiment we must stay rigidly on that line, but we must refer to the landmarks on the phase diagram map to understand what is happening. Of course, should water be allowed to evaporate from the liquid during mixing, or condensation on a cooled slab be incorporated, the consequences can be ascertained in the obvious manner (see §5.13).
The first increments of the ZnO powder to be added to the acid liquid only dissolve, since this part of the mixing line is in the Liquid phase field. Whether or not zinc is complexed by phosphate in solution is irrelevant and the ionic constitution of the liquid does not require consideration in the reaction scheme. It is, however, quite improper to say that “primary phosphate” exists in solution.4 This simple dissolution continues until the liquidus is reached, somewhere between points P1 and P2. Most of this part of the addition is, of course, represented by the zinc content of the liquid as supplied commercially. However, this cannot be taken too far as solid would appear on cooling, or even on slight evaporation of water from the liquid.
P1 and P2 represent the compositions of two liquids. But since they are both aqueous solutions in the same system they are, of course, miscible. This means that all points lying between them on the P1 – P2 composition line represent only single liquids – not two phases – which may be viewed as the result of mixing different proportions of the two solutions represented by P1 and P2. (This also applies in equivalent terms to the lines between P1 and H2O, and between P2 and P3.)
•5.5 Stage 2
Continuing along the acid – ZnO mixing line, crossing the liquidus takes us into the Liquid + β2-phase field (Fig. 5.6). The precipitation of “secondary” phosphate trihydrate therefore commences. We can be sure that the phase formed first is indeed β2 because we see from the diagram that it is stable in contact with the liquid – while that liquid continues to exist. Conversely, there is no phase field with the description.ζ + Liq; the ZnO is therefore unstable with respect to that liquid, hence it dissolves. The reaction may be written as:
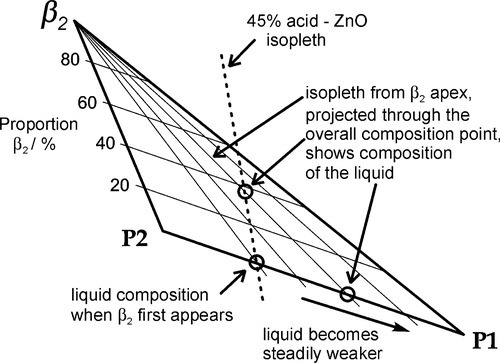
As further ZnO dissolves, so more β2 is precipitated, but now the acid concentration of the liquid declines as a result. This may seem a little odd, but note that the molar ratio of H3PO4 : H2O is 1:7 at about 44% mass% acid, so that removing these components in the ratio 1:2 (according to reaction 5.3) must result in acid dilution. This can be seen graphically by drawing a line from the β2 apex through the overall composition point (on the dashed mixing line), extended to cut the liquidus between P1 and P2. This intersection point moves towards P1 as the amount of ZnO increases until, of course, it coincides with P1. The liquid then corresponds to about 20 – 25 mass% acid, while the overall composition lies on the β2 – P1 line. In addition, as this phase field is crossed, the proportion of liquid in the mixture is decreasing from 100 mass% at the liquidus to about 42 mass% at the β2 – P1 line, which is therefore being treated as a composition line itself.
•5.6 Stage 3
When the β2 – P1 line is crossed (Fig. 5.7) by the addition of more ZnO, the first step must be the production of the “tertiary” zinc phosphate tetrahydrate:
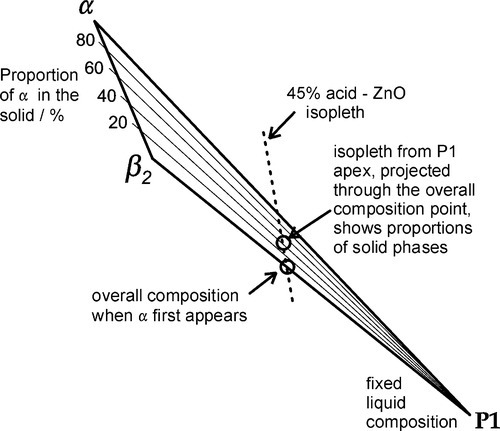
(i.e. precisely the same as the overall reaction 5.1 – it is only now that it actually happens directly). The acid of the liquid must therefore made more dilute because the effective concentration of the acid required (91.6% acid, reaction 5.1) is greater than that of the P1 liquid itself, i.e. about 22% acid. The new liquid would therefore correspond to a composition on the H2O side of point P1 on the liquidus. But clearly β2 is now unstable in contact with that more dilute liquid, since it does not appear in a phase field adjacent to such a composition.
The only stable solid phase for contact with liquids of compositions lying between P1 and the H2O apex is α. Hence, after such further addition of powder, some of the β2 already precipitated must decompose, enough to cause the liquid composition to return to that corresponding to P1. A disproportionation reaction therefore occurs:
The ‘liberated’ liquid is thus essentially 52% ‘free’ acid, and indeed this replenishes the diluted acid liquid, bringing its composition back to that composition corresponding to P1.
An alternative view of the changes occurring as the α + β2 + Liquid phase field is crossed, still following the dashed mixing line from 45% acid, is to write the overall reaction as:
It must be emphasized that the small amount of liquid produced overall in this process must correspond exactly to P1, whose composition does not change during this stage of the process. The fact that some liquid is produced is shown by the mixing line (dashed in Fig. 5.7) being at a slight angle to the α-β2 side of the composition triangle. This is clearly true for any such isopleth passing through this field since they must by definition pivot on the ZnO apex.
•5.7 Stage 4
Once the β2-phase has been used up, the overall composition enters the α + Liquid phase field (in the triangle marked by α – P1 – H2O) (Fig. 5.8). Continuing to follow the mixing line, direct precipitation of the α-phase now continues according to reaction 5.4. Now, since the molar ratio of H3PO4 : H2O consumed in this process is 2:1, the liquid is yet again diluted, the composition moving quickly towards the H2O apex, following the liquidus. In fact, the liquidus cannot quite meet that apex because ZnO and “tertiary” zinc orthophosphate are not completely insoluble, but the distinction is too fine to be drawn in this diagram.
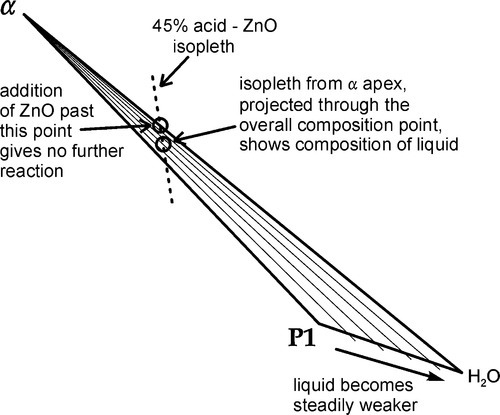
Nevertheless, once the α-H2O boundary is crossed, there is no further reaction. Any subsequent addition of ZnO remains as unreacted core in the reaction product matrix, which is of course what is required for the composite structure to have the necessary mechanical properties.
The overall setting process is therefore first the precipitation of β2, then α with the concomitant conversion of β2, followed by the formation of α alone. This is summarized in Fig. 5.9.
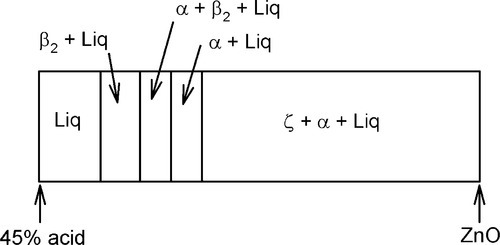
•5.8 Kinetic limitation
The above scheme is based essentially on the assumption in the thought experiment of the attainment of equilibrium after each infinitesimal increment of ZnO. However, the disproportionation reaction 5.5 proceeds only slowly, even in contact with quite dilute acid (composition to the right of P1), and it is this that constitutes the basis of the final setting reaction of the clinical cement: the slow release of acid delays the completion of the formation of α-phase. In other words, the formation of β2 represents a reservoir for a proportion of the acid that is only slowly made available for final reaction. Without this kinetic limitation, the cement would set far too quickly to be of any clinical use. This mechanism also accounts for the long-continuing low pH measured on the surface of apparently set cement, because the remaining liquid is continually replenished with free acid. Completion of the reaction would be indicated by a tendency for the pH to rise again from its intermediate, dynamic quasi-equilibrium value when the β2 ⇒ α and ζ ⇒ α reactions are proceeding at equivalent rates.
•5.9 Stronger acid
To return to the thought experiment: if the acid liquid is sufficiently concentrated initially, that is, greater than about 58 mass% H3PO4, β1 will be the first solid precipitated during the addition of powder:
We can see this by choosing as acid a concentration corresponding to about />
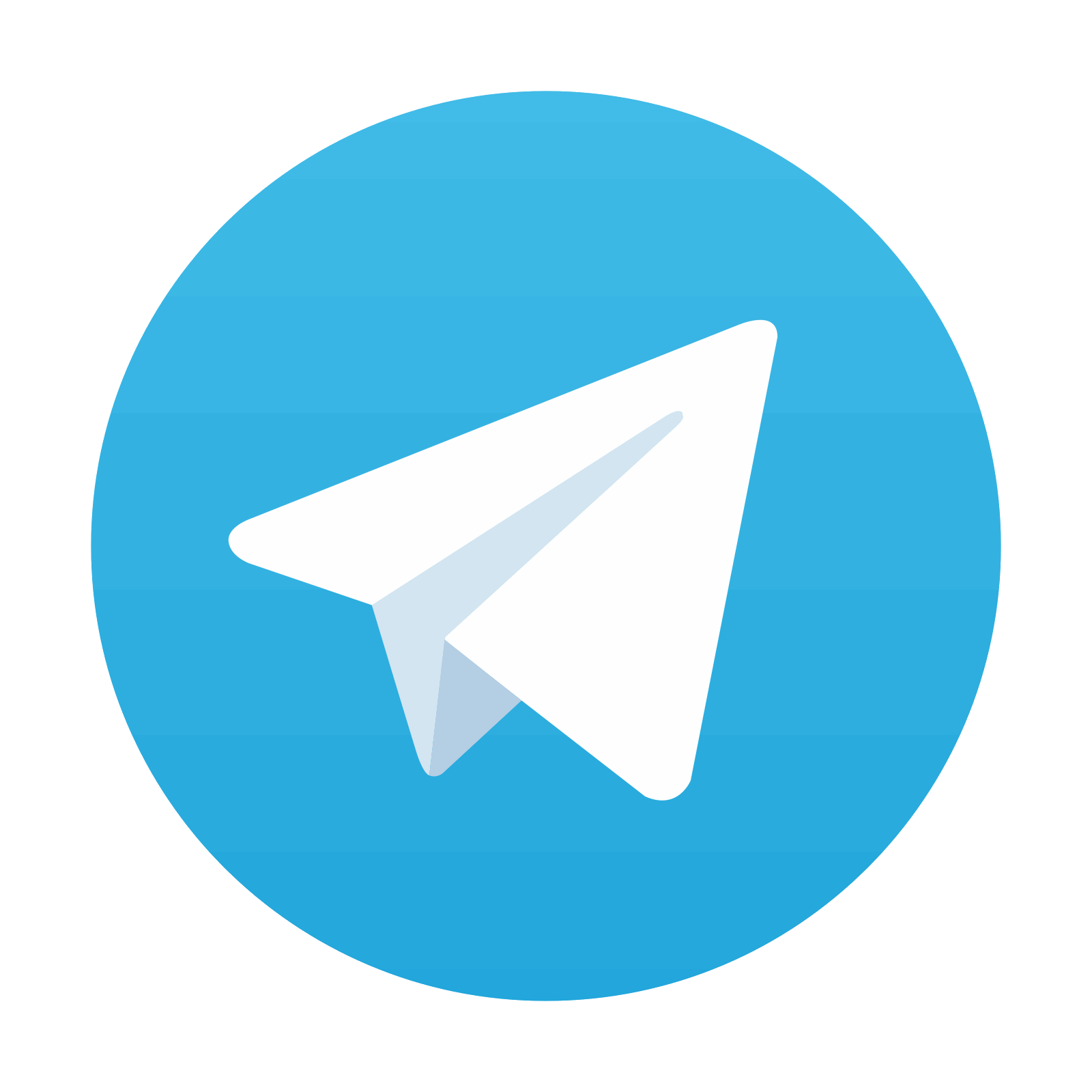
Stay updated, free dental videos. Join our Telegram channel

VIDEdental - Online dental courses
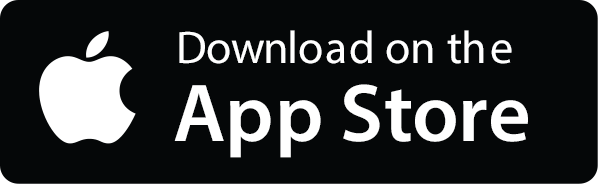
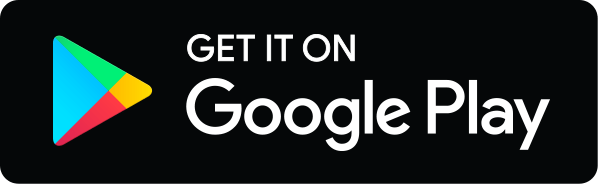