Chapter 8
Regenerative Periodontal Therapy
INTRODUCTION
The aim of periodontal regeneration is complete restoration of the attachment apparatus. Specific components such as periodontal ligament, cementum, and alveolar bone must contribute to this biological process for regeneration to occur. Periodontal ligament cells, osteoblasts, and cementoblasts must be in the regenerative site to achieve a clinically acceptable result. Regeneration, in contrast to conventional periodontal resective surgery, achieves pocket elimination/reduction and attachment gain by a biological process and not repair. Furthermore, pocket elimination procedures result in attachment loss that may cause root caries, sensitivity, and an esthetically compromised dentition. Meanwhile, regenerative surgical procedures alter factors during the wound healing process that shift repair to restoration of architecture. Ideally, a patient who suffered from periodontitis should have a periodontium comparable to a periodontally healthy individual following a regenerative treatment.
Regenerative periodontal procedures require biomaterials that lead to regeneration of the periodontal ligament (PDL) and new attachment. Earlier periodontal procedures for regeneration included bone and/or bone substitutes alone. A variety of barrier membranes to contain the graft material were later added to the procedure. In addition, graft materials or carrier materials (scaffold) are necessary depending on the purpose, e.g., space to be maintained at the site, and/or delivery of growth/regeneration factors. An ideal grafting material should deliver the regenerative signals in an optimal manner to stimulate a cellular response.
The specific predictability of periodontal regenerative procedures has focused on its indications. In general, the morphology of the bone defect limits the outcome. The procedures aim first to eliminate or reduce periodontal pockets, second to restore the lost alveolar process with regeneration of PDL and bone, and last to regenerate a functional attachment apparatus, ideally to periodontally healthy levels (Schallhorn, 1977). In general, indications for a regenerative procedure are deep intraosseous defects or cases in which osseous resective surgery is contraindicated because a substantial amount of supporting bone has been lost. In localized aggressive periodontitis, a regenerative approach should be considered first because osseous resective therapy can cause more harm. Regeneration minimizes post surgical clinical attachment loss.
Periodontal regeneration can be achieved by various techniques. These methods can be generally classified into grafting alone, guided tissue regeneration (graft and membrane), and growth factor stimulated regeneration. Grafting procedures alone can involve several material types including autogenous, allograft, xenograft, and alloplasts (Box 8.1). A variety of materials have the potential for periodontal stimulation for bone grafts. Materials commonly used for that purpose are osteogenic (bone graft material that has viable cells that can produce bone), osteoinductive (bone graft material that contains factors that may stimulate new bone growth), and osteoconductive (bone graft material that does not have any bone stimulatory factors in it but rather acts as a scaffold for bone growth).
Regeneration associated with grafting may result in long junctional epithelium because new PDL, cementum, and connective tissue (CT) attachment may not be established. However, acceptable clinical healing, including pocket reduction and attachment gain, may occur. Initial efforts to achieve guided tissue regeneration (GTR) aim to regenerate PDL and the rest of the periodontium by isolating the defect with barrier membranes and/or grafting to maintain space so that the regeneration can take place. Guided tissue regeneration is based on the exclusion of connective tissue and epithelium in favor of PDL regeneration, and following establishment of a new attachment. Thus, GTR is the purposeful selection of cell types that repopulate at the wound with the intention of directing the healing tissue composition. Barrier materials include natural absorbable polymers such as collagen (Types I, II, III, IV) and collagen and glucoseaminoglycan (GAG) copolymer; synthetic absorbable polymers such as polylactic acid and polyglycolic acid; fibrin; synthetic non-resorbable polymer polytetraflouroethylene (PTFE); synthetic ceramics such as calcium phosphate; and natural bone mineral.
Recently, the stimulation of periodontal regeneration with growth factors has become an effective and predictable technique. Based on biological enhancement of wound healing, these molecules produce a true histological regeneration. Growth/regeneration factors and differentiation factors such as enamel matrix proteins (amelogenin), polypeptide mitogens such as bone morphogenic protein -2(BMP-2), growth factors such as platelet derived growth factor (PDGF), and a combination of growth factors such as platelet rich plasma (PRP) are being used to stimulate regeneration. These molecules augment and/or stimulate the natural healing response and include stimulatory effects on angiogenesis, cellular differentiation, cellular proliferation, celular ingrowth, and extracellular matrix biosynthesis, respectively. The stimulation by signaling molecules (growth factors) has improved the predictability in periodontal defects.
PERIODONTAL REGENERATIVE TECHNIQUES
The contemporary periodontal approach should eliminate the etiology and correct the defects by returning the periodontium to healthier levels. Periodontal regenerative procedures are necessary to achieve this goal (Figure 8.1). The advantage to periodontal regeneration not only includes attachment gain, but also provides benefits in areas with local or dental anatomical factors such as root concavities or where deep interproximal periodontal breakdown occurs. In addition, esthetics may be enhanced.
Guided Tissue Regeneration
Guided tissue regeneration is based on several key concepts such as new attachment, reattachment, regeneration, and repair. The term guided tissue regeneration was first used when a new connective tissue attachment was demonstrated with human histology by using a ePTFE membrane (non-resorbable) (Gottlow et al., 1986). Additional terminology ncludes new attachment (union of connective tissue or epithelium with root surface that has been deprived of its original attachment), reattachment (reunion of epithelium or connective tissue with root surfaces and bone such as occurs after ncision or injury), regeneration (a histological term describing the reconstruction of an injured part), and repair (healing of a wound that is not fully restored to its original form and function).
Exclusion of epithelium is a critical component of barrier membrane regeneration. Epithelial cells migrate three to five times faster than PDL cells. As a result, the invasion by the epithelium does not allow PDL to regenerate. The first study showing that histological new attachment was possible used a Millipore filter to exclude epithelium (Nyman et al., 1982a,b), which showed that space creation by a membrane and/or graft is effective in periodontal regeneration. Cellular response investigations indicate that wound healing originates from the PDL (Aukhil et al., 1986). The peak mitotic activity of PDL occurs at approximately three weeks (Nyman, 1982). The importance of the PDL cell response in GTR is also critical to cementum formation, which allows PDL fibers to attach. Therefore, cementum formation is considered the rate-limiting step in periodontal regeneration (Caton et al., 1987).
Several factors are important in the outcome of guided tissue regeneration, including periodontal defect morphology, remaining periodontium, and practitioner skill. Guided tissue regeneration is technique-sensitive because the surgical area is narrow and soft tissue management is difficult. Meanwhile, flap design must consider primary closure of the surgical site to achieve full coverage of the regenerative membrane. An optimal amount of tissue must be retained in the flap design; typically, intrasulcular incisions are preferred. Releasing incisions also may be used as necessary (anatomy permitting) because access for thorough debridement of the surgical site from granulation tissue is important for success. Vertical incisions may also facilitate proper flap coverage of the membrane and graft material placement.
Following adequate flap elevation, the root surface must be debrided and deposits removed for new attachment. The consensus is that root conditioning with chemical agents is not recommended because no clear benefits have been established (Mariotti, 2003). Root surface mechanical preparation is essential in conjunction with hand instrumentation (curettes) or sonic or ultrasonic hand pieces.
Membrane adaptation around the tooth and onto the defect must be optimal to favor PDL growth without any significant epithelial downgrowth. Any significant irregularity of the bone that may affect the position of the membrane should be eliminated with conservative osteoplasty. As necessary, the membrane must be trimmed accordingly, adapted, and sutured around the tooth. Flap adaptation should be maximized around the tooth and onto the membrane for proper coverage. In addition, the flap should be passive onto the surgical site to prevent any dehiscence during the healing period. Suture material such as ePTFE or polyglycolic acid material should be chosen to close the site because these retain the least amount of plaque. Finally, a periodontal dressing may be used to secure the surgical area, especially from food impaction or trauma. Postoperative care is important and patients are typically prescribed an antibiotic, anti-inflammatory, and antibacterial rinse during the initial healing period.
Figure 8.1. Extraction and ridge augmentation in the anterior maxilla with bone graft and resorbable membrane by guided tissue regeneration principles, implant placement, and final restoration. A, Fractured upper left central incisor (pus is noticeable on the gingival margin) and missing upper right central incisor. B, Upon flap reflection, extraction and degranulation were completed. Buccal plates for both sockets were entirely resorbed. C, Bone graft and resorbable membranes are in place. The membranes are critical for epithelial exclusion in favor of proper bone regrowth in the area. D, Radiographic evaluation prior to implant placement, approximately four months after bone grafting, reveals uniform bone formation. E, Complete clinical bone regeneration achieved approximately four months later. F, Implant placement at bone regenerated site to replace upper right central incisor and upper left central incisor. G, Final implant-supported fixed restorations for upper right central incisor and upper left central incisor with a desirable esthetic result.
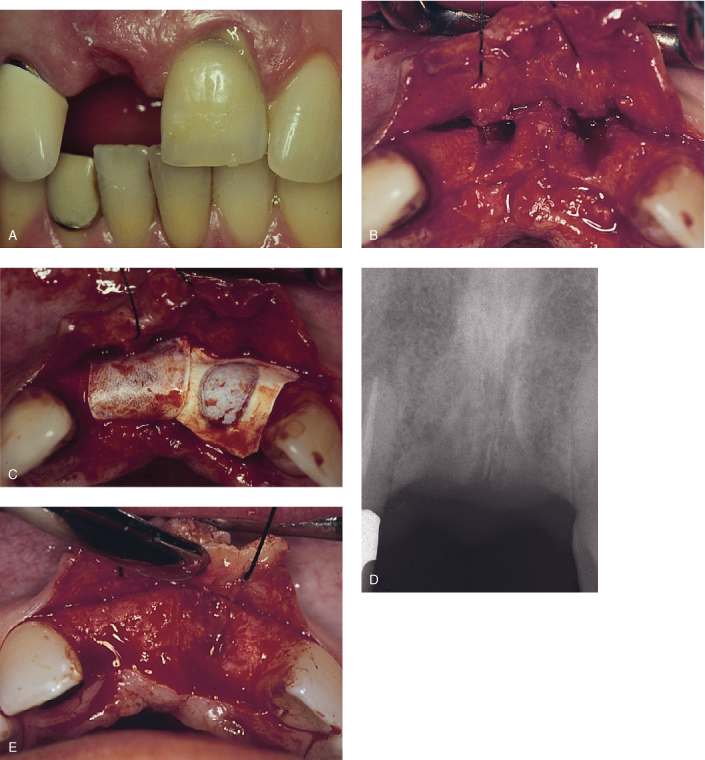
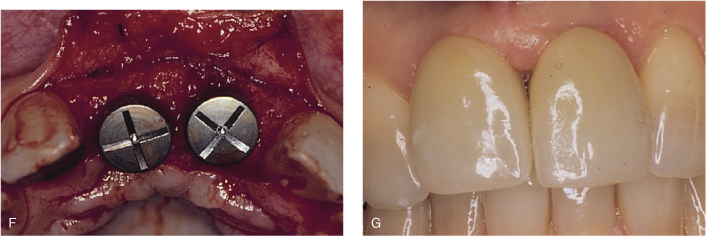
Pontoriero et al. (1987, 1995) demonstrated in a series of studies that GTR is a predictable procedure for Class II furcations (Hamp classification) compared to open flap debridement. However, that predictability decreased significantly for Class III furcation-involved molars. For maxillary molars, buccal furcations are more predictable than the interproximal area. Meanwhile, a six month re-entry study showed that results were enhanced with GTR with a non-resorbable membrane and DFDBA, compared to GTR alone. The GTR and DFDBA combination produced more defect fill and pocket depth reduction and a greater gain in the clinical attachment level (Anderegg et al., 1991).
Depending on the number of bone wall defects, GTR showed 95% fill of three-wall, 82% fill of two-wall, and 39% fill of one-wall. Overall, a 73% defect fill was achieved (Cortellini et al., 1993a,b). In terms of defect fill, the areas of deepest probing depth demonstrated the greatest pocket depth (PD) reduction and clinical attachment level (CAL) gain (Selvig et al., 1993). Long-term results indicate that CAL gains were maintained four to five years following GTR (Gottlow et al., 1992). Good oral hygiene and patient compliance were found to be critical in long-term stability of GTR procedures (Cortellini et al., 1994).
Signaling Molecules in Periodontal Tissue Regeneration
There has been mounting evidence that the choice of graft material may play a significant role in altering the wound healing, from repair to regeneration. The analysis of autograft or allograft has found that signaling molecules contained in the material may stimulate regeneration. The molecular bases of these factors have been identified, characterized, and produced in large quantities. Several signaling molecules are currently being investigated with preclinical and clinical studies.
Enamel Matrix Proteins
Enamel matrix protein (EMP) or enamel matrix derivative (EMD) is a commercially available regenerative material. Amelogenin is the main constituent (95%) of this group of proteins; the remaining approximate 5% are other less characterized proteins. These proteins, especially amelogenin, are thought to be involved in acellular cementum, PDL, and alveolar bone development. The EMD that is currently available is isolated from porcine tooth buds (Hammarström, 1997). These porcine-derived proteins are very similar to proteins expressed during human enamelogenesis, but there are small genetic differences (Gestrelius et al., 1997a,b). In vitro studies with enamel proteins suggested that periodontal ligament cells’ RNA expression is stimulated. Enamel proteins improved PDL cell function, particularly their effects on cell metabolism (Barkana et al., 2007). In addition, enamel proteins contributed to new blood vessel formation (Schlueter et al., 2007).
Osteoblast growth and differentiation, another critical component for periodontal regeneration, has been shown to be enhanced by EMD. In vitro studies indicate that EMD contributes to osteoblastic activity on human osteoblasts. In addition, it limits bone resorptive/osteoclastic activity. Moreover, important osteoblastic activity markers were enhanced at two and three weeks, an indication of continued biological activity (Galli et al., 2006).
Further evaluation of enamel matrix proteins for periodontal regeneration in a mouse model demonstrated that new cellular cementum-like tissue formed along EMP-treated root slices (Song et al., 2007). Meanwhile, in order to understand the effects on soft tissues, human gingival fibroblast cells were treated with EMD and the cell cycle was assessed. The results suggested that EMD induced mitogenic activity for gingival fibroblasts, which may explain better soft tissue healing clinically (Zeldich et al., 2007).
Clinical investigations have demonstrated multiple benefits of the regeneration of periodontal support with EMD. The use of EMD in patients with lower molar Class II buccal furcation defects resulted in reduced horizontal probing at 14 months post-surgery as compared to those treated with traditional GTR (Hoffmann et al., 2006). Another trial evaluating contralateral randomized infrabony defects using EMD vs. GTR with resorbable membrane demonstrated similar improvements. These improvements in periodontal parameters were maintained up to eight years (Sculean et al., 2006). In addition numerous clinical case series have indicated positive results with EMD (Cortellini and Tonetti, 2007). A localized bone defect associated with a palatal groove was successfully treated with EMD. This particular treatment is limited to a case report with 8 mm clinical attachment gain and 2 mm of residual probing depth one year post surgically. However, it is viable evidence of the success for regenerative treatment in such complex lesions with EMD (Zuchelli et al., 2006).
Recombinant Human Platelet-derived Growth Factor
Recombinant human platelet-derived growth factor (rhPDGF) is a polypeptide growth factor which has been shown to stimulate periodontal regeneration. rhPDGF has specific effects on wound healing properties, including the regeneration of periodontium. rhPDGF is especially a strong mitogen for mesenchymal cells. Indeed, it has been shown that human osteoblastic cells produce PDGF. On a molecular basis, pdgf-a gene expression results in the PDGF protein. PDGF has PDGF-AA (acidic) and PDGF-BB (basic) variant forms. While human osteoblasts respond to PDGF-BB uniformly, there is an inconsistent response to PDGF-AA (Zhang et al., 1991).
Originally platelet-derived growth factor (PDGF) and insulin-like growth factor-I (IGF-I) were found, in combination, to synergistically upregulate DNA and protein synthesis in osteoblasts. Additional in vivo findings indicated that this effect would be achieved on soft tissue. Early studies suggested and evaluated the synergistic effects of PDGF and IGF-1 in periodontal defects in canine and human models with naturally occurring periodontitis. In preclinical models, rhPDGF-BB and rhIGF-1 in a carrier gel were applied on the root surfaces of periodontitis-affected teeth in conjunction with an open flap debridement procedure. Histological analysis of control carrier gel specimens indicated the presence of long junctional epithelium without any clear new attachment in two weeks. As expected, PDGF/IGF-1-treated sites demonstrated considerable new attachment as well as new bone highly populated with osteoblasts and new cementum. Significant osteoblast presence in PDGF-applied specimens was evidence of bone regeneration that perhaps would continue beyond two weeks.
Similarly, a carrier gel, with or without radioactively labeled rhPDGF-BB and rhIGF-1, was applied during periodontal surgery on beagles with naturally occurring periodontal disease. This experimental design made it possible to show the clearance rate of rhPDGF-BB and rhIGF-1 protein. The results revealed that the half-life of the molecules at the site of application was three hours for IGF-I and up to 4.2 hours for PDGF-BB. Almost all of the radioactively labeled protein was cleared after 96 hours, and two weeks later no radioactivity was detected. However, twice as much radioactive material was bound at the surgical site in the experimental group, which indicated that PDGF and IGF-1 bind on the target cells, which would trigger biological activity during periodontal regeneration. Histomorphometric analyses on two-and five-week specimens demonstrated five-to ten-fold increases in new bone and cementum as compared to controls (Lynch et al., 1991).
Meanwhile, the mitogenic effect of natural platelet-derived growth factor (nPDGF) on periodontal ligament fibroblast-like cells was investigated by using radioactive isotope incorporation in DNA. nPDGF stimulated approximately three times more DNA synthesis compared to the control and two times more compared to fibroblast growth factor (Blom et al., 1994). In vitro or laboratory findings are ultimately important for clinical practice to provide a proof of principle basis for biological activity. In that regard, in order for PDGF to optimally stimulate periodontal regeneration, the PDGF protein must bind its cellular receptor in periodontal tissues. Moreover, periodontal ligament cells may have the ability to differentiate into cementoblasts and/or osteoblasts with this stimulation.
Although PDGF-BB is well established as a key stimulator for periodontal regeneration in vitro and in vivo, further clinical studies were required to validate its effects on human periodontal defects. A split-mouth study was carried out with rhPDGF-BB and rhIGF-I in a carrier gel. Re-entry surgical procedures were performed to assess bone fill six to nine months following treatment. Results demonstrated that 2.08 mm of new vertical bone height gain and 42.3% osseous defect fill in rhPDGF-BB/rhIGF-1-treated defects occurred in comparison to 0.75 mm and 18.5% for t/>
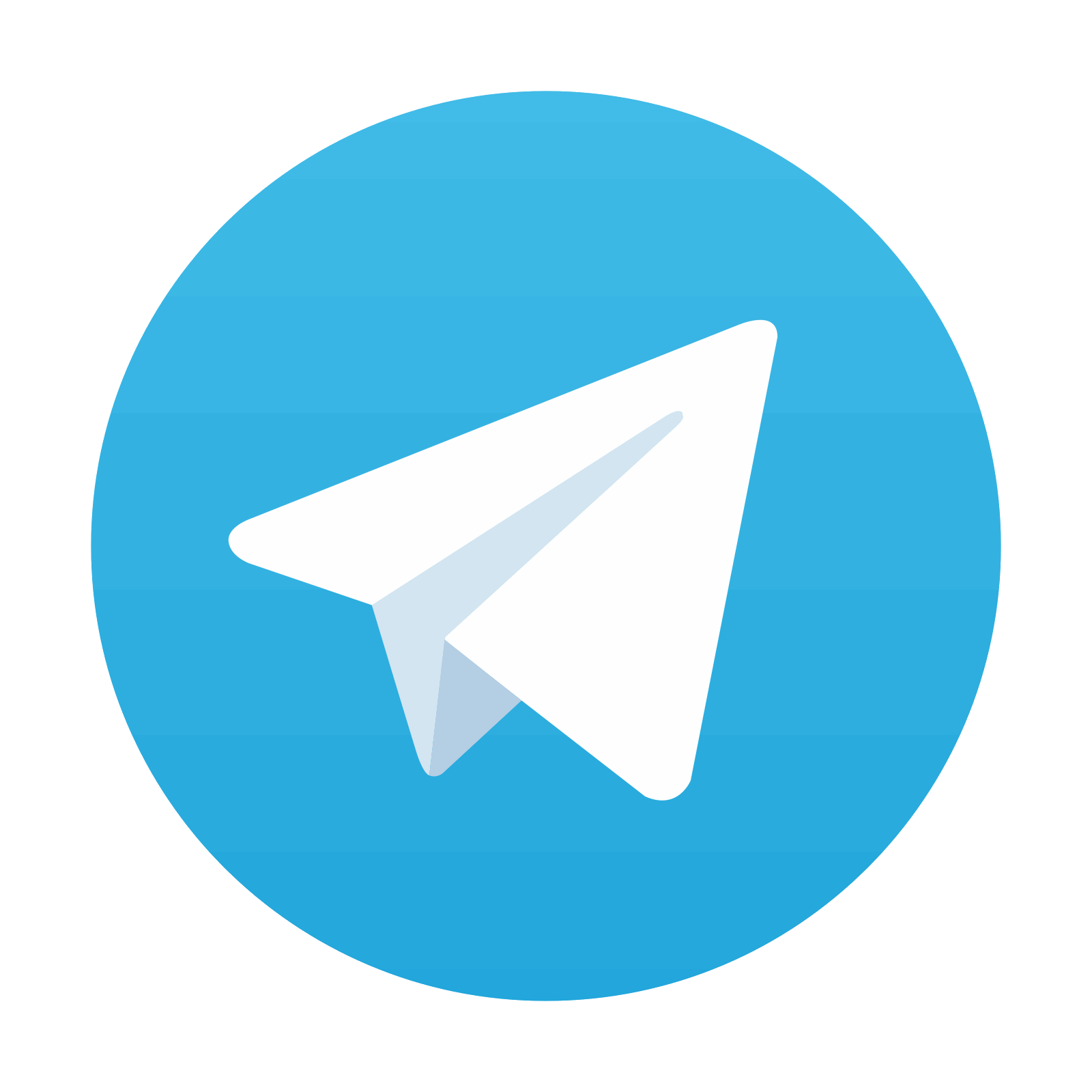
Stay updated, free dental videos. Join our Telegram channel

VIDEdental - Online dental courses
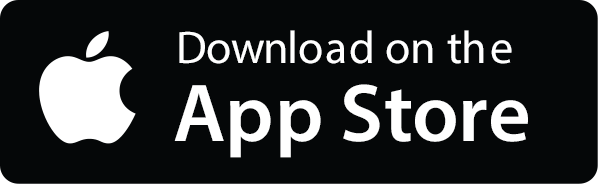
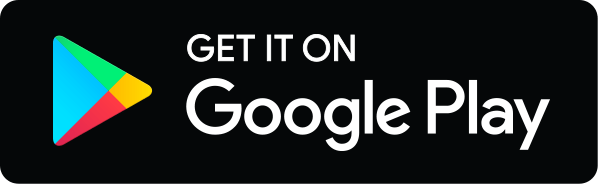