Chapter 7. Salivary glands, saliva and salivation
Physiology and biochemistry of salivary glands and saliva80
Overview
In producing saliva, the exocrine salivary glands are essential for the maintenance of oral health. Although it is over 99% water, the small content of other elements (such as bacteriocidal agents and growth factors) allows the saliva to undertake many functions. There are both major and minor salivary glands, and serous, mucous or mixed glands; secretion is under the control of the autonomic nervous system. The primary saliva produced by the parenchymal cells undergoes modification within striated ducts as it passes along the duct system. Reduction in salivary flow in older patients, often as a side-effect of drugs, can give rise to dry mouth (xerostomia).
Learning objectives
You should:
• know the formation, composition and functions of saliva and how its secretion is controlled
• be able to describe the gross anatomy and relationships of the major salivary glands and the situation of the groups of minor salivary glands
• understand the histology of the salivary glands both in terms of the parenchymal cells (mucous and serous) and the nature of the duct system, and be able to appreciate the differences between the three pairs of major salivary glands
• be aware of how such knowledge is relevant to the clinical situation.
Salivary glands are compound, tubuloacinar, merocrine, exocrine glands whose ducts open into the oral cavity. The term compound refers to the fact that a salivary gland has more than one tubule entering the main duct; tubuloacinar describes the morphology of the secreting cells; merocrine indicates that only the secretion of the cell is released; exocrine describes a gland that secretes fluid on to a free surface. The many functions of saliva include:
• lubrication for mastication, swallowing and speech
• bringing substances in solution for taste
• acting as a buffer to maintain the integrity of enamel
• limiting the activity of bacteria
• promoting the health of the oral mucosa.
Salivary glands may be classified according to size (major and minor) and/or the types of secretion (mucous, serous or mixed). The three, paired, major salivary glands are the parotid, the submandibular and the sublingual glands. The numerous minor salivary glands are scattered throughout the oral mucosa and include the labial, buccal, palatoglossal, palatal and lingual glands.
Gross anatomy
Parotid gland
The parotid gland is serous and is the largest of the major salivary glands. It occupies the region between the ramus of the mandible and the mastoid process. The parotid is pyramidal in shape; its apex extends beyond the angle of the mandible and the base is closely related to the external acoustic meatus. The deep surface of the gland rests anteriorly on the ramus and masseter. The gland is surrounded by an unyielding tough fibrous capsule, the parotid capsule. The parotid duct appears at the anterior border of the gland and passes horizontally across the masseter muscle before piercing the buccinator to terminate in the oral cavity opposite the maxillary second molar. Lying with the duct on the masseter may be an accessory parotid gland.
Within the parotid gland are found the external carotid artery, the retromandibular vein and the facial nerve. Branches of the facial nerve are seen emerging from the anterior and inferior margins of the gland. Appearing at the superior border of the gland are the superficial temporal vessels and the auriculotemporal nerve. From the inferior border of the gland may be seen the anterior and posterior branches of the retromandibular vein. Lymph nodes are also associated with the parotid gland.
The parasympathetic innervation of the parotid gland is from the lesser petrosal branch of the glossopharyngeal nerve. The preganglionic fibres synapse in the otic ganglion, and postganglionic fibres reach the gland by travelling with the auriculotemporal branch of the mandibular nerve.
Submandibular gland
The submandibular gland is a mixed gland but is primarily serous. A large part it (the superficial part) is visible just beneath the inferior border of the mandible, where it lies on the mylohyoid muscle. The gland has an important relationship with the mylohyoid muscle, wrapping around the free posterior border (not unlike the letter C). This gives rise to the smaller deep portion of the gland that lies on the hyoglossus muscle. The submandibular duct appears from the deep part of the gland and wraps around the lingual nerve as it crosses the hyoglossus muscle to terminate on the sublingual papilla in the floor of the mouth.
Sublingual gland
The sublingual gland is the smallest of the three major salivary glands. It is a mixed gland but has a preponderance of mucous elements. The sublingual gland is not a single unit like the parotid and submandibular glands, but is made up of one large segment (the major sublingual gland) with a main duct that either joins the submandibular duct or drains directly on to the sublingual papilla, and a group of 8–30 mixed, minor salivary glands, each having its own duct system emptying into the sublingual fold in the floor of the mouth. The major sublingual gland is a mixed gland with a preponderance of mucous elements. The sublingual gland lies between the hyoglossus and mylohyoid muscles and lies against the sublingual fossa of the mandible.
The parasympathetic innervation of both the submandibular and sublingual glands is from the chorda tympani branch of the facial nerve. Preganglionic fibres are carried via the lingual nerve to the submandibular ganglion. Postganglionic fibres pass from this ganglion to the submandibular and sublingual glands.
Physiology and biochemistry of salivary glands and saliva
Saliva has a number of major functions, being important in mastication, swallowing, digestion, maintenance of oral hard and soft tissues, control of oral microbial population, and voice and speech articulation. Lubrication of the oral cavity is important in that it enhances the movement of the tongue and lips, and aids in cleansing the oral cavity of food debris and bacteria. Mastication, bolus formation and swallowing depend on a moist, lubricated oral mucosa and fluid to wet the bolus. Digestive enzymes are present in saliva and aid in the initial process of digestion. Saliva has a protective function, maintaining an effective barrier to external insults. Saliva calcium and phosphorus are in supersaturated concentrations in the saliva and play a role in the remineralization of the teeth. Also a neutral pH in the oral cavity is maintained by the buffering capacity of the saliva.
Composition of saliva
About 99% is water, with the remaining 1% being made up of ions and organic constituents. It is most often hypotonic when compared with plasma; however, it has the ability to be isotonic and even hypertonic under physiological control mechanisms. The important ions in saliva are the cations Na + and K +, and the anions Cl − and bicarbonate (HCO 3−). Other electrolytes present in saliva are calcium phosphate, fluoride, thiocyanate, magnesium sulphate and iodine. Saliva is derived from blood plasma but is not an ultrafiltrate of plasma.
Organic constituents
The organic constituents consist of proteins, carbohydrates, lipids and small organic molecules.
Proteins and glycoproteins
Saliva contains a variety of proteins and glycoproteins, including the serum proteins γ-globulins, albumin and α/β globulins. The proteins synthesized in the glands are:
• IgA (analogous to serum proteins)
• enzymes, including amylase, lysozyme, peroxidase, kallikrein and small amounts of many others (e.g. acid phosphatase, RNAase, cholinesterase, lipase)
• glycoproteins that contribute to viscosity of saliva, enhancing and facilitating its lubricating and agglutinating properties
• various small N-containing compounds.
In general, the parotid gland will synthesize more protein than glycoprotein, so parotid saliva has a lower carbohydrate content, whereas the submandibular and sublingual glands synthesize and secrete greater amounts of glycoprotein than protein, and saliva from these two glands is higher in carbohydrate content. This is observed histologically, where the parotid gland contains high levels of secretory granules (protein-rich) but no secretory droplets (mucin-rich), which are present in the submandibular and sublingual glands.
The function of the salivary proteins can be related to their structural features; in general they facilitate the lubricating properties of the saliva, essential for healthy mucosal tissue and antimicrobial protection. The presence of the enzymes such as amylase highlights the digestive role played by saliva, whereby complex starch present in food can be broken down (amylase hydrolyses α1–4 glycosidic linkages) prior to the food bolus entering the stomach. Lysozyme, acid phosphatases and peroxidases may well facilitate the protective role saliva plays in maintaining a balanced oral microflora, as these enzymes have antimicrobial properties. Lysozyme functions by cleaving β-N-acetylmuramic acid residues in bacterial cell walls and aggregates bacterial cells in suspension. It can also enhance the activity of immunoglobulins. Salivary peroxidase catalyses the reaction of bacterial metabolic products, hydrogen peroxide with salivary thiocyanate to oxidized derivatives. Salivary carbonic anhydrase increases the buffering capacity of saliva by producing bicarbonate. The secretory immunoglobulin IgA is produced as a specific response to contact with an antigen. It is synthesized by immune cells and translocated to the surface by epithelial cells. Lactoferrin binds ferric iron (Fe 3+), an essential microbial nutrient, and therefore demonstrates some antibacterial activity (nutritional immunity) but can have direct bactericidal effects on some micro-organisms.
Salivary mucins are a large family of glycoproteins existing with differing oligosaccharide chains and a protein core. Two main mucin subfamilies have been identified: namely, MG1 (high molecular weight and high carbohydrate content) and MG2 (lower molecular weight, and lower carbohydrate content). These mucins form superstructures through electrostatic bonds and formation of covalent disulphide bonds. The main functions of salivary mucins relate to:
• lubrication (due to the high negative charge of salivary mucin, superstructures adopt an expanded structure aiding lubrication)
• hydration (as mucins exhibit negative charges that help bind water)
• pellicle formation and remineralization (MG1 helps in binding Ca 2+ and hydroxyapatite)
• facilitating the direct removal of bacteria (MG2 can interact with bacteria).
Saliva contains a number of small molecular weight proteins that are generally phosphorylated and non-glycosylated. These include the proline-rich peptides, which have a negatively charged amino terminal that is phosphorylated and a positively charged carboxy terminal. These function by inhibiting calcium phosphate crystal growth; they may also have a role in remineralization of enamel. They also exhibit selective interaction with oral bacteria and other pellicle proteins, demonstrating a role in pellicle formation. Statherin, a tyrosine-rich protein, has a negative amino terminal (phosphorylated) and hydrophobic carboxy terminal. These molecules function by maintaining supersaturated levels of Ca 2+ and PO 4 whilst inhibiting mineral formation in the salivary glands and oral cavity. The histatins are histidine-rich proteins and are inhibitors of Candida albicans and Streptococcus mutans growth in the oral cavity.
Growth factors
Growth factors (epidermal growth factor and nerve growth factor) and other regulatory peptides are also present in saliva, though the precise role of these has not been determined with certainty.
Formation of saliva
As mentioned earlier, saliva is mostly a hypotonic secretion. Its formation is dependent upon the stimuli mediated by both the parasympathetic and sympathetic parts of the autonomic nervous system. Parasympathetic cholinergic stimuli provide the principal stimulus for fluid secretion; however, it is most likely that under reflex control mechanisms, cholinergic, adrenergic and peptidergic neurotransmitters are involved in the secretion of saliva.
Primary stage of salivary secretion
Saliva is formed in the acinar cells of the glands and is delivered into the mouth through a series of ductal trees. Salivary secretion is essentially a two-stage process. The primary stage of salivary secretion results in the formation of an isotonic primary secretion by the acinar cells; this is later rendered hypotonic by the removal of Na + and Cl − as it flows through the ductal system with little loss of fluid volume. When the parasympathetic nerves are stimulated, acetylcholine (ACh) binds to the muscarinic ACh receptors and causes an increase in inositol trisphosphate (IP 3) levels. This rise in IP 3 levels leads to an increase in Ca 2+ release from intracellular stores and, in turn, these higher Ca 2+ levels lead to an increase of fluid secretion from the cells. The mechanisms brought into play by this increase in Ca 2+ are as follows:
• Cl −, which is concentrated within the acinar cells, is released across the apical membrane of the acinar cell.
• This leads in turn to a secretion of Na +.
• The combined NaCl secretion takes water across the cells by osmosis.
Water crosses the epithelium by two possible routes, moving:
• through the tight junctions between the cells via paracellular transport
• through the acinar cells through both the apical and basolateral membranes via transcellular transport.
Second stage of salivary secretion
The second stage of salivary secretion involves the modification of the isotonic saliva secreted by the acini into the hypotonic saliva secreted from the salivary ducts into the mouth. This ductal modification of the primary, acinar-derived isotonic solution is effected by apical Cl − and Na + channels, along with Cl −/HCO 3− and Na +/H + exchanges. These allow movement of Na + and Cl − along a concentration gradient into the striated duct cells and then drive them out of the cell across the basolateral membrane. As the striated duct cells are impermeable to water, there is no osmotically driven reabsorption of water and so the saliva in the duct becomes hypotonic. Since the fluid secretory process in the acinar cells has a greater capacity than the electrolyte reabsorption process in the ductal cells, there can be large composition changes of the saliva entering the mouth. At low, resting flows, saliva moves slowly through the ducts and the striated epithelium cells are able to modify the composition of the saliva substantially by absorbing Na + and Cl −. However, at high, stimulated flows the saliva passes rapidly through the ducts with much less alteration of electrolyte concentrations. Thus, the electrolyte concentrations of saliva at high rates of flow are more similar to the primary saliva concentrations produced by the acinar cells.
Bicarbonate secretion
Salivary bicarbonate ions (HCO 3−) are important in buffering plaque acid within the mouth. HCO 3− can pass through Cl − channels and so are secreted along with Na + and Cl − into the ductal lumen by the acinar cells and possibly the intercalated duct cells close to the acinar cells. At low salivary flow rates HCO 3− is reabsorbed by the striated duct cells and so very little will get into the mouth; however, at high flow rates there is less reabsorption of HCO 3− and therefore higher concentrations reach the mouth when needed during eating.
Protein secretion
Whilst the secretions of water and electrolytes are a secretory modality called ‘hydrokinetic’, the secretion of proteins is termed ‘proteokinetic’. Both these functions do not occur synchronously but are influenced by which nerves are firing, the impulse frequency and also the type of cell that is being stimulated. In general, the bulk of the fluid flow is generated by parasympathetic nerve activity. The amount of protein secreted tends to be low when impulse frequencies are low and higher when the stimulus is greater. The flow of saliva is usually less with sympathetic nerve stimulation but it does cause a greater concentration of proteins and this can lead to greater outputs for short periods of time. It is thought that sympathetic activity is usually superimposed on parasympathetic activity rather than in isolation and thus produces synergistic effects on flow and protein outputs. Proteins are secreted in two possible ways:
• Firstly, by ‘exocytosis’ of pre-packaged proteins from secretory granules in specialized exocrine cells
• Secondly, by a process called ‘constitutive secretion’ from the movement of Golgi-derived vesicles to the surface of the cells in all glandular cells.
It has been shown that similar-looking cells in different glands produce quite different proteins, and similar mucins may be differently glycosylated in different glands.
Reflex activity
Reflexes are automatic, predictable, reproducible and goal-directed responses to stimuli. Most are innate and almost all involve the central nervous system. Salivary secretion is dependent on reflex activity. Resting flows are present throughout the day and night and keep the mouth and oropharynx moist, lubricated and protected. However, in human beings large increases in secretion over short periods of time are seen during eating and these increases are attributed, in varying degrees, to stimulation of a number of sensory receptors, including chemoreceptors involved in gustation and olfaction, mechanoreceptors and nociceptors.
The parotid, submandibular, sublingual and minor salivary glands all contribute to what is termed whole-mouth saliva. Not only does the secretion from the different glands vary in composition and volume to a given stimulus, but also the saliva produced by a single gland is variable. Therefore, the mixed whole-mouth saliva can vary considerably in its volume and composition depending on the type, amplitude and duration of the stimuli applied. The control of salivation depends on reflex nerve impulses. These reflexes involve afferent limbs, salivary nuclei within the medulla, and efferent limbs comprising both the sympathetic and parasympathetic secretomotor nerves supplying the various glands. Eating is the main cause of an increase of salivary flow above that of resting levels of flow. A variety of receptors are stimulated before, during and following the ingestion of food and drink; amongst these are gustatory, masticatory, olfactory, psychic, visual, thermoreceptive and possibly nociceptive. Inevitably, the afferent inputs during normal eating will comprise combinations of all these stimuli in variable amounts and lead to a complex reflex response. However, most studies have been done on the individual reflexes and very few on the combinations of inputs and their responses. There are also a number of reflex responses in which salivary secretion occurs, which are not normally associated with eating, such as nausea, vomiting and pain.
Whole-mouth saliva flow rates vary from resting with a mean of 0.3 ± 0.22 ml min −1. When stimulated, the whole-mouth salivary flow will rise to a mean of 1.7 ± 2.1 ml min −1. The daily flow rates lie between 500 and 1000 ml per day. The contribution to the whole-mouth saliva made by the different glands varies according to whether the gland is at rest or stimulated:
• The parotid gland provides only 20% of the saliva when rested and as much as 50% when stimulated.
• The submandibular gland provides over 65% of resting whole-mouth saliva, but only 30% of stimulated saliva.
• The sublingual and minor salivary glands supply the remaining saliva equally in both rest and stimulated conditions.
Gustatory-salivary reflex
Stimulation of gustatory receptors, mainly found in the taste buds, leads to the reflex secretion of saliva (gustatory-salivary reflex). All five basic tastes (salt, sour, sweet, bitter and umami) will cause a reflex salivary secretion. The volume and composition of saliva depend on the quality of the stimulus. There is common agreement that sour stimuli will evoke a maximal secretory response from most salivary glands. Lower concentrations of acid, along with all other basic gustatory stimuli, give variable degrees of salivary responses; however, all are considerably smaller than the maximum flow seen with, for instance, 5% citric acid. Very few foods contain an acid concentration as high as 5% citric acid; it therefore follows that concentrations as high as these cannot be regarded as normal (physiological) gustatory stimuli. The gustatory stimulus that evokes the greatest salivary response is sour, followed by umami, salt, sweet and bitter. Contrary to some suggestions, fats do not cause a salivary reflex response, suggesting that there are no specific fat receptors on the taste receptor cells in the taste buds.
The transduction of a chemical stimulus to an electrical event within the taste bud receptor cell leads to the initiation of action potentials, which are transmitted by the afferent limb of the reflex to the salivary nuclei. It is thought to be the pattern of these impulses and the type and location of the gustatory receptors, and not the perception of the taste, that lead to a reflex salivary flow. The perception must be a parallel response.
There is evidence that, not only does the individual gustatory stimulus produce different volumes of saliva, but also it can produce saliva with different overall compositions unrelated to the fact that the rate of salivary flow through the ducts affects the concentrations of some electrolytes. In some animals sweet stimuli have been shown to produce low flows of parotid saliva with a high protein content and, in human beings, it has been shown that salt stimuli produce parotid secretions higher in proteins than do other basic stimuli at the same flows.
Masticatory-salivary reflex
There is now considerable evidence that mastication causes a reflex salivary secretion (masticatory-salivary reflex). When one chews on one side of the mouth, there is greater flow of saliva from the parotid gland on that side than from the gland on the opposite non-chewing side. It appears that ‘each gland seems to be most intimately associated with the receptors on its own side’ (Lashley 1916). It has been shown that the output of saliva from the parotid gland is directly proportional to the masticatory forces and there is now substantial evidence that intra-oral mechanoreceptors, and in particular periodontal ligament mechanoreceptors, contribute to this reflex. The role of mucosal mechanoreceptors in the reflex cannot be excluded, as the reflex is still present in edentulous subjects and flow is considerably reduced when the oral mucosa underlying the dentures is anaesthetized with a topical anaesthetic ointment.
Olfactory-salivary reflex
Since the classical work of Pavlov in the late 1920s, on the conditioned reflex, it has been assumed that the smell of food causes salivation in human beings (olfactory-salivary reflex). Many textbooks state the existence of an olfactory-salivary reflex and significant increases in whole-mouth salivary flow have been recorded in response to olfactory stimulation. Over the years there has been some confusion as to whether olfactory stimuli cause secretion in all the major glands. Recently it has been shown that in human beings a true olfactory-parotid salivary reflex does not exist when normal pleasant food odours are presented to the olfactory epithelium. When the same pleasant food odours are presented to the olfactory epithelium and flow recordings made from the submandibular/sublingual glands, there are, however, significant increases in flow of saliva. This suggests that, whilst olfactory stimuli do cause a reflex secretion of saliva in human beings, this reflex involves the submandibular/sublingual glands and not the main parotid glands.
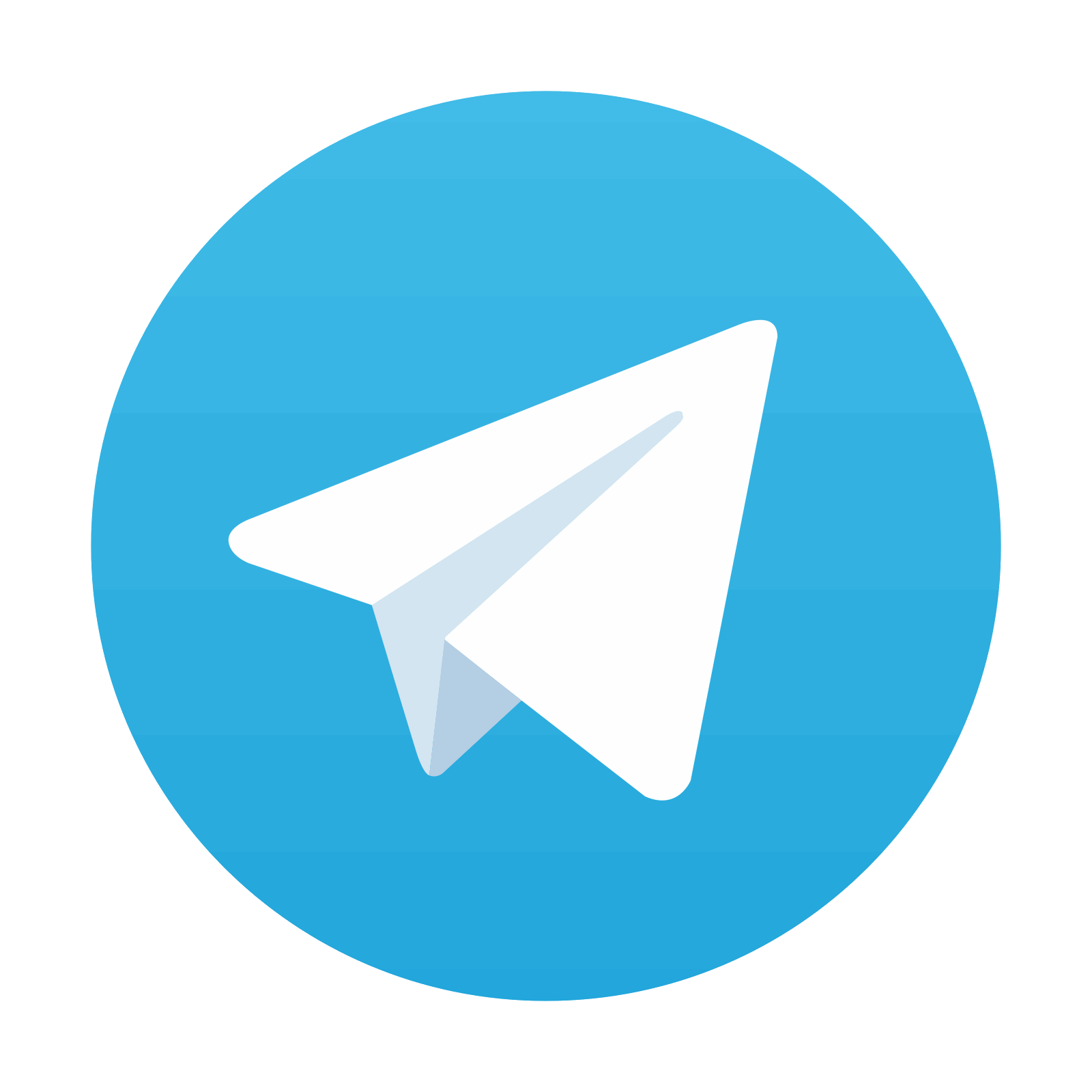
Stay updated, free dental videos. Join our Telegram channel

VIDEdental - Online dental courses
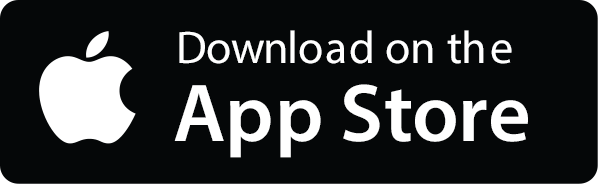
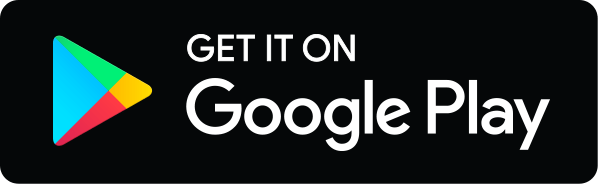