Chapter 5. Tongue, flavour, thermoreception and speech
Taste53
Overview
The tongue is a muscular organ which, in addition to moving the food bolus around the mouth during chewing (mastication) and into the pharynx during swallowing (deglutition), is involved in the selection of food for ingestion (via taste) and in speech. This chapter also covers the role of the tongue in gustation and the sensation of flavours (thus including olfaction as well as taste) and thermoreception in the oral cavity as a whole.
Learning objectives
You should:
• have a detailed knowledge of the macroscopic anatomy of the tongue and be able to relate this to function
• have a good knowledge of the physiological mechanisms underlying the sensation of flavours, about thermoreception in the oral cavity, and about the role of the tongue and mouth in speech.
Introduction
The tongue is a muscular organ with its base attached to the floor of the mouth. It is attached to the inner surface of the mandible near the midline and gains support below from the hyoid bone. It functions in mastication, swallowing and speech and carries out important sensory functions, particularly those of taste. The lymphoid material contained in its posterior third has a protective role.
Ventral surface
The ventral surface of the tongue (the inferior surface), related to the floor of the mouth, is covered by a thin lining of non-keratinized mucous membrane that is tightly bound down to the underlying muscles. In the midline, extending onto the floor of the mouth, lies the lingual frenum. Rarely, this extends across the floor of the mouth to be attached to the mandibular alveolus. Such an overdeveloped lingual frenum (ankyloglossa) may restrict movements of the tongue (tongue-tie). Lateral to the frenum lie irregular, fringed folds: the fimbriated folds. Also visible through the mucosa are the deep lingual veins.
Dorsum
The dorsum of the tongue may be subdivided into the anterior two-thirds (palatal part) and the posterior third (pharyngeal part). The junction of the palatal and pharyngeal parts is marked by a shallow V-shaped groove, the sulcus terminalis. The angle (or ‘V’) of the sulcus terminalis is directed posteriorly. In the midline, near the angle, may be seen a small pit called the foramen caecum. This is the primordial site of development of the thyroid gland. The mucosa of the palatal part of the tongue is partly keratinized and is characterized by the abundance of papillae. The most conspicuous papillae on the palatal surface of the tongue are the circumvallate papillae, which lie immediately in front of the sulcus terminalis. The pharyngeal surface of the tongue is covered with large rounded nodules termed the lingual follicles. These follicles are composed of lymphatic tissue, collectively forming the lingual tonsil. The posterior part of the tongue slopes towards the epiglottis, where three folds of mucous membrane are seen: the median and lateral glosso-epiglottic folds. The anterior pillars of the fauces (the palatoglossal arches) extend from the soft palate to the sides of the tongue near the circumvallate papillae.
The dorsum of the tongue is covered with numerous whitish, conical elevations: the filiform papillae, which are keratinized. Interspersed between the filiform papillae are isolated reddish prominences, the fungiform papillae. These are non-keratinized and contain taste buds. The fungiform papillae are most numerous at the tip of the tongue. The 10–15 circumvallate papillae are considerably larger than either the filiform or fungiform papillae. They lie immediately in front of the sulcus terminalis, do not project beyond the surface of the tongue and are surrounded by a circular ‘trench’. The surface of the posterior third of the tongue lying behind the sulcus terminalis is non-keratinized and is covered by a number of smooth elevations produced by underlying lymphoid tissue. Foliate papillae appear as a series of parallel, slit-like folds of mucosa on each lateral border of the tongue, near the attachment of the palatoglossal fold (anterior pillar of the fauces). The foliate papillae are of variable length in humans and are the vestige of large papillae found in many other mammals.
The histological appearance of the mucosa of the tongue is described on page 240.
Muscles
The tongue is composed of intrinsic and extrinsic muscles:
• The intrinsic muscles of the tongue are restricted to the substance of the tongue and change its shape.
• The extrinsic muscles arise outside the tongue and are responsible for bodily movement of the tongue.
Intrinsic muscles
The intrinsic muscles of the tongue can be divided into three fibre groups: transverse, longitudinal and vertical. Rarely can these three groups be distinguished in dissections, but their interlacing gives the tongue its characteristic appearance in cross-section.
• The transverse fibres arise from a sheet of connective tissue called the lingual septum, running longitudinally through the midline of the tongue. These transverse fibres pass laterally from the septum to intercalate with fibres of the other groups of intrinsic muscles.
• The longitudinal fibres may be subdivided into upper and lower groups: the superior and inferior longitudinal muscles of the tongue.
• The vertical fibres pass directly between the upper and lower surfaces, particularly at the lateral borders of the tongue.
Contraction of the vertical fibres would make the tongue thinner (and wider). Contraction of the longitudinal fibres would shorten (and thicken) the tongue. Contraction of the transverse fibres would narrow (and widen) the tongue. The intrinsic muscles receive their motor innervation from the hypoglossal (XII th) cranial nerve.
Extrinsic muscles
The extrinsic muscles of the tongue arise from the skull and hyoid bone and thence spread into the body of the tongue. The extrinsic musculature is composed of four groups of muscles: genioglossus, hyoglossus, styloglossus and palatoglossus.
Genioglossus
The genioglossus muscle arises from the superior genial tubercle on the medial surface of the body of the mandible. At this level, the two genioglossus muscles cannot be readily separated. As the muscles enter the tongue, a thin strip of connective tissue intervenes between the right and left genioglossus muscles. The bulk of the fibres fan out into the body of the tongue but the superior fibres pass upwards and anteriorly to the tip of the tongue, and some of its inferior fibres insert onto the body of the hyoid bone. The genioglossus muscle is mainly a protractor and depressor of the tongue.
Hyoglossus
The hyoglossus muscle originates from the superior border of the greater horn of the hyoid bone and passes vertically upwards into the tongue. Its function is to depress the tongue. At its origin, the hyoglossus muscle is separated from the attachment of the middle constrictor muscle of the pharynx beneath by the lingual artery.
Styloglossus
Each styloglossus muscle arises from the anterior surface of the styloid process of the temporal bone, from which the muscle runs downwards and forwards to enter the tongue below the insertion of the palatoglossus muscle. At this point, its fibres intercalate with the fibres of the hyoglossus before continuing forwards towards the tip of the tongue. The styloglossus muscle is a retractor of the tongue.
Palatoglossus
Each palatoglossus muscle arises from the aponeurosis of the soft palate and descends to the tongue in the anterior pillar of the fauces, whence its fibres intercalate with the transverse fibres of the tongue. The action of the palatoglossus muscles is to raise the tongue in order to narrow the transverse diameter of the oropharyngeal isthmus. The extrinsic muscles of the tongue are innervated by the hypoglossal nerve (XIIth cranial nerve) (except for the palatoglossus, which is innervated by the cranial part of the accessory nerve (XIIth cranial nerve) via the pharyngeal plexus).
Blood supply
The main source of the blood supply to the tongue is the lingual artery. This is a branch of the external carotid artery in the neck. The artery passes into the floor of the mouth deep to the hyoglossus muscle (superficial to the superior constrictor muscle), where it is accompanied by the glossopharyngeal nerve. Dorsal lingual branches supply the dorsum of the tongue and a sublingual branch the floor of the mouth. The terminal branch is the deep lingual artery found running near the lingual frenum on the ventral surface of the tongue. Lingual veins are variable. There is usually a dorsal lingual vein draining the dorsum of the tongue and this becomes the lingual vein that drains into the internal jugular vein in the neck. The deep lingual vein on the ventral surface of the tongue joins the sublingual vein from the sublingual salivary gland to become ‘the vein accompanying the hypoglossal nerve’ (this also drains into the internal jugular).
Lymphatic drainage
The lymphatic drainage of the tongue is mainly into the submandibular nodes (the lateral lymph vessels draining ipsilaterally but more central vessels draining both ipsilaterally and contralaterally). Lymph from the tip of the tongue drains into the submental nodes. Lymphatics from the posterior third of the tongue drain into the deep cervical chain of nodes (including jugulodigastric nodes) (again the lateral lymph vessels drain ipsilaterally but more central vessels drain both ipsilaterally and contralaterally).
Sensory innervation
Three distinct nerve fields can be recognized on the dorsum of the tongue:
• The anterior part of the tongue, in front of the circumvallate papillae, is supplied by the lingual branch of the mandibular division of the trigeminal nerve (V3 cranial nerve) However, its accompanying chorda tympani fibres from the nervus intermedius part of the facial nerve (VIIth cranial nerve) are those associated with the perception of taste. Note that the chorda tympani nerve joins the lingual nerve in the infratemporal fossa and deep to the inferior head of the lateral pterygoid muscle.
• Behind, and including the circumvallate papillae, the tongue is supplied primarily by the glossopharyngeal nerve (IXth cranial nerve) providing both general sensation and taste.
• A small area on the posterior part of the tongue around the epiglottis is supplied by the vagus nerve (Xth cranial nerve) via its superior laryngeal branch.
The mucosa on the ventral surface of the tongue is supplied by the lingual nerve.
The development of the tongue is considered on page 104.
Taste
When we eat and drink we experience a sensation that is commonly called taste, yet eating is a multi-sensory experience involving not only the chemical senses of taste (gustation) and smell (olfaction), but also the senses of texture and touch (mechanoreception), temperature (thermoreception), light (vision), pain (nociception) and even sound (audition). Just think of the combinations of experiences — touch, fizziness, coolness, acidity, colour and exquisite smells, as well as the sound of the popping of the cork — when drinking champagne. Those who like spices in their food derive pleasure from the stimulation of receptors in the sensation of pain (nociceptors). These are stimulated by chemicals found in the common spices, such as chilli peppers, and the resulting sensation is referred to as the common chemical sense.
Taste buds
Taste (gustation) refers to the sensation experienced when chemicals come into contact with the gustatory end organs, the taste buds. Taste buds are embedded in the stratified epithelium of the tongue, soft palate, pharynx, larynx and epiglottis, and are unevenly distributed around these regions. They are innervated by the facial, glossopharyngeal and vagus nerves. The lingual taste buds are associated with three of the four types of papillae (fungiform, foliate and circumvallate), whilst those associated with the other regions of the mouth are found on the smooth epithelial surfaces.
In human beings, the number of taste buds varies from person to person with, on average, a range of 2000–5000, but it can be as low as 500 and as high as 20 000 with no significant age or gender differences. The papillae in the different regions of the tongue have distinctive shapes and characteristic numbers of taste buds associated with them, as well as distinctive innervations related to their position on the tongue.
• Scattered over the main body of the tongue are approximately 200 small, mushroom-shaped, fungiform papillae, which have on average three taste buds each.
• The larger foliate papillae are found on the back and sides of the tongue. They comprise up to nine folds of epithelium and as many as 600 taste buds each.
• About 10–15 larger mushroom-shaped, circumvallate papillae, each surrounded by a circular trough, lie on the back of the tongue in a V-shaped formation. The circumvallate papillae have on average 250 taste buds each.
Each taste bud is connected at its base by terminals of sensory nerve fibres. The nerve supply for most of the taste buds on the soft palate and on the anterior two-thirds of the tongue comes from the chorda tympani branch of the facial nerve, so called because its route to the brain stem passes close to the tympanic membrane in the ear. The glossopharyngeal nerve innervates the taste buds on the posterior third of the tongue and the back of the mouth, whilst branches of the vagus nerve innervate taste buds in the pharynx and epiglottis.
Each taste bud is made up of up to 50–150 neuroepithelial cells arranged like the segments of an orange in a compact pear-shaped structure (intragemmal cells). The taste bud complex is about 70 μm high and 40 μm in diameter, and has a small 2–10 μm opening in the epithelium surface, called the taste pore, which allows direct contact between the chemicals dissolved in the saliva and the small tips of some of the neuroepithelial receptor cells within the taste bud. The exposed parts of the receptor cells are made up of many long, corrugated folds in the membrane called microvilli that provide a large surface area for contact with the chemicals dissolved in the saliva. Saliva is essential for normal taste, as it acts as the solvent for the chemicals as well as a transport medium for the chemicals to reach the receptors. There is a layer of saliva that extends into the taste pore and which constantly bathes the receptor tips. The dissolved chemicals diffuse through this thin layer to reach the microvilli.
There is general agreement that there are four types of intragemmal cell: basal cells (stem cells) that give rise to the receptor cells, type I (dark) cells, intermediate and type II (light) cells. It has been suggested that these different types represent the different stages in the life cycle of the taste receptor cell.
The taste bud complex is an extremely dynamic system in which there is a rapid turnover of receptor cells. The lifespan of an individual receptor cell is approximately 10 days, with cells being continually born through the division of epithelial stem cells (basal cells) within the bud, maturing, performing their gustatory function and then eventually dying.
The receptor cells do not in themselves have an axon or nerve fibre, but the base of each cell has specialized regions that look like terminals of nerve fibres. The cytoplasm at the base of the cell is packed with small vesicles filled with a chemical transmitter substance (possibly serotonin or vasoactive intestinal peptide), which is released when the potential inside becomes more positive (becomes depolarized). In most cases, the depolarization leads to an action potential followed by an increase in intracellular Ca 2+ within the cell and the subsequent release of the neurotransmitter. In close association with these regions are the endings of the sensory (intragemmal) nerve fibres that make a synaptic-like connection with the receptor cells. The released neurotransmitter elicits generator potentials and hence action potentials in this primary afferent neurone, thereby transmitting impulses into the central nervous system. Each taste bud is innervated by more than one nerve fibre and each single nerve fibre can innervate a number of receptor cells, taste buds and even papillae. This means that there is a high degree of convergence of inputs from taste buds on to the sensory nerve fibres. As there is a rapid turnover of receptor cells, there are also constantly changing connections between the cells and the nerve fibres. The nerves are continually sprouting new processes, forming new synapses with young cells and retracting synaptic connections with dying cells. It has been calculated that, at any one time, fewer than one-third of the cells in a single taste bud are fully innervated. It has been shown that an intact nerve supply is necessary for the normal survival and function of taste buds. If the nerve supply is cut or damaged, the taste buds degenerate and slough off. Following nerve regeneration, the taste buds reappear and can return to normal function.
Transduction mechanisms
Since Aristotle (384–322 bc), there have been attempts to categorize taste into primary or basic qualities of taste. Although many hundreds of different chemicals can stimulate activity in taste receptor cells, the four basic qualities of taste (salt, sour, sweet and bitter) have stood the test of time. A fifth basic quality of taste has been recently identified. This is referred to as umami (delicious taste), which is associated with the amino acid glutamate and some nucleotides.
Gustatory receptor cells have a dual role, namely that of detecting both nutrients and toxins. Because of this, they have to be able to respond, both individually and collectively, to a wide variety of chemicals. These chemicals can be simple ions, such as sodium ions (salt) and hydrogen ions (sour), or the more complex compounds that give the sensations of sweet (glucose), bitter (quinine) and umami (monosodium glutamate).
The transduction mechanisms that convert the chemical stimuli into electrical events in the receptor cell are numerous, varied and sometimes complex. There does not appear to be a unique mechanism for each of the basic tastes; each seems to use several different mechanisms. There may even be similarities of mechanisms for different basic tastes. The way in which we perceive many subtle tastes and distinguish different compounds of the same basic taste category could be explained by the specificity and multiplicity of these mechanisms. However, we do know that simple Na + salts depolarize taste cells by Na + influx through channels in the apical and lateral cell membrane. Similar channels may be involved in the detection of H + ions, although the transduction mechanism for this ion shows a marked species variability. Transduction of more complex molecules such as sugars and bitter substances frequently involves membrane receptors linked to G-proteins and second messengers, such as cyclic adenosine monophosphate (cAMP) and inositol trisphosphate (IP 3/diacylglycerol (DAG)) that gate ion channels and cause depolarization and action potentials to be initiated. Some artificial sweeteners are known to cause depolarization by modulating ligand-gated ion channels directly. The response to glutamate (umami) is thought to involve N-methyl-D-aspartic acid (NMDA) and metabotropic glutamate receptors, similar to those found in the brain. In most cases, the resultant depolarization leads to an action potential in the receptor cell that in turn is followed by an increase in intracellular Ca 2+ and the release of the neurotransmitter from the base of the cell associated with the intragemmal nerve ending. Recordings, using tiny electrodes, from individual nerve fibres innervating the taste buds in anaesthetized animals reveal that one does not experience a burst of impulses only when a solution of just one of the basic taste substances is dripped on to the appropriate taste bud or buds. Such selectivity of response is very rare. Most nerves respond to two or more of the basic taste stimuli, the magnitude of the response varying from one taste substance to another (the so-called taste profile). This means that the activity of a single gustatory fibre does not provide unambiguous information to the brain about the quality and intensity of the stimulus. At some point the brain must perform a comparison between the activities in several different nerve fibres in order to determine what the taste actually is.
Afferent gustatory neurones
The primary afferent gustatory neurones have their first synaptic connection within the nucleus of the tractus solitarius (NTS) and it is here that approximately 82% of the neurones respond to three or four basic taste stimuli. There is also convergence here between gustatory and thermoreceptor inputs, with many neurones responding to cooling of the tongue, as well as to chemical stimuli. The second-order neurones in the NTS then project to the most medial part of the ventroposterior medial nucleus of the thalamus. There is a further projection to the brain-stem reticular formation, the parabrachial nuclei and the cranial nerve nuclei involved in the reflexes associated with gestation. The thalamic neurones project to the primary gustatory cortex, which includes an area just anterior to the somatosensory area for the tongue, as well as to the nearby frontal operculum and anterior insular secondary cortex. There is evidence for a secondary gustatory area in the orbitofrontal cortex; studies in primates suggest that neurones at these higher levels of the gustatory pathway respond more selectively, with 25% of the neurones in the primary cortex and 74% of the neurones in the secondary cortex responding to a single basic gustatory stimulus. Neurones in the orbitofrontal cortex that respond to gustatory stimuli are seen to be modulated by hunger and inputs are reduced when appetite is satiated. The orbitofrontal cortex receives inputs not only from the gustatory neurones, but also from the olfactory, visual and somatosensory pathways, and neurones, have been shown to respond to two or more modalities (touch, vision, smell, taste). This area is thought to be involved in the learning of associations between stimuli: for instance, the association of the taste, smell, texture and sight of food substances, all of which are important in the appreciation of what is termed ‘flavour’.
Several textbooks and detailed reviews on taste have, in the past, suggested that different areas of the tongue are more sensitive to the different basic tastes. These so-called tongue maps are now thought to be incorrect, their origin lying in a mistranslation of the early 20th-century work of Hänig. His work referred to thresholds of the basic tastes and not to the exclusive nature of the loci. Subsequent misinterpretations have led to the impression that the tip of the tongue detects sweet, the sides of the tongue detect sour and salt, and the back of the tongue detects bitter tastes. This is clearly not true.
Common chemical sense
The common chemical sense is the sensation caused by stimulation of epithelial or mucosal-free nerve endings by potentially harmful chemicals. The evidence suggests that free nerve endings are polymodal nociceptors that respond to a variety of different modalities of stimuli, such as mechanical, thermal and noxious. In the mouth, the major nerve that contributes to this sense is the trigeminal nerve. This innervates almost all regions of the mouth, the tongue, the hard and soft palate, the mucosa of the lips, and cheek. Free nerve endings are found throughout the oral cavity and amongst the chemicals that are known to stimulate these receptors (besides noxious, damaging chemicals) are alcohol, menthol, peppermint, capsaicin and piperine, the last two found in chilli peppers and black pepper respectively.
Olfaction
The human olfactory organ (the olfactory epithelium) comprises a sheet of cells 100–200 μm thick, situated high in the back of the nasal cavity and on a thin bony partition called the central septum of the nasal passage. This system responds to volatile, airborne molecules that make contact with the olfactory epithelium with the in-out air flow during normal nasal breathing. There is a turbulent air flow in this region because of the turbinate bones in the side walls of the nose (the conchae), and this leads to the odour molecules being distributed over the receptor sheet in an irregular pattern.
Olfactory epithelium
/>
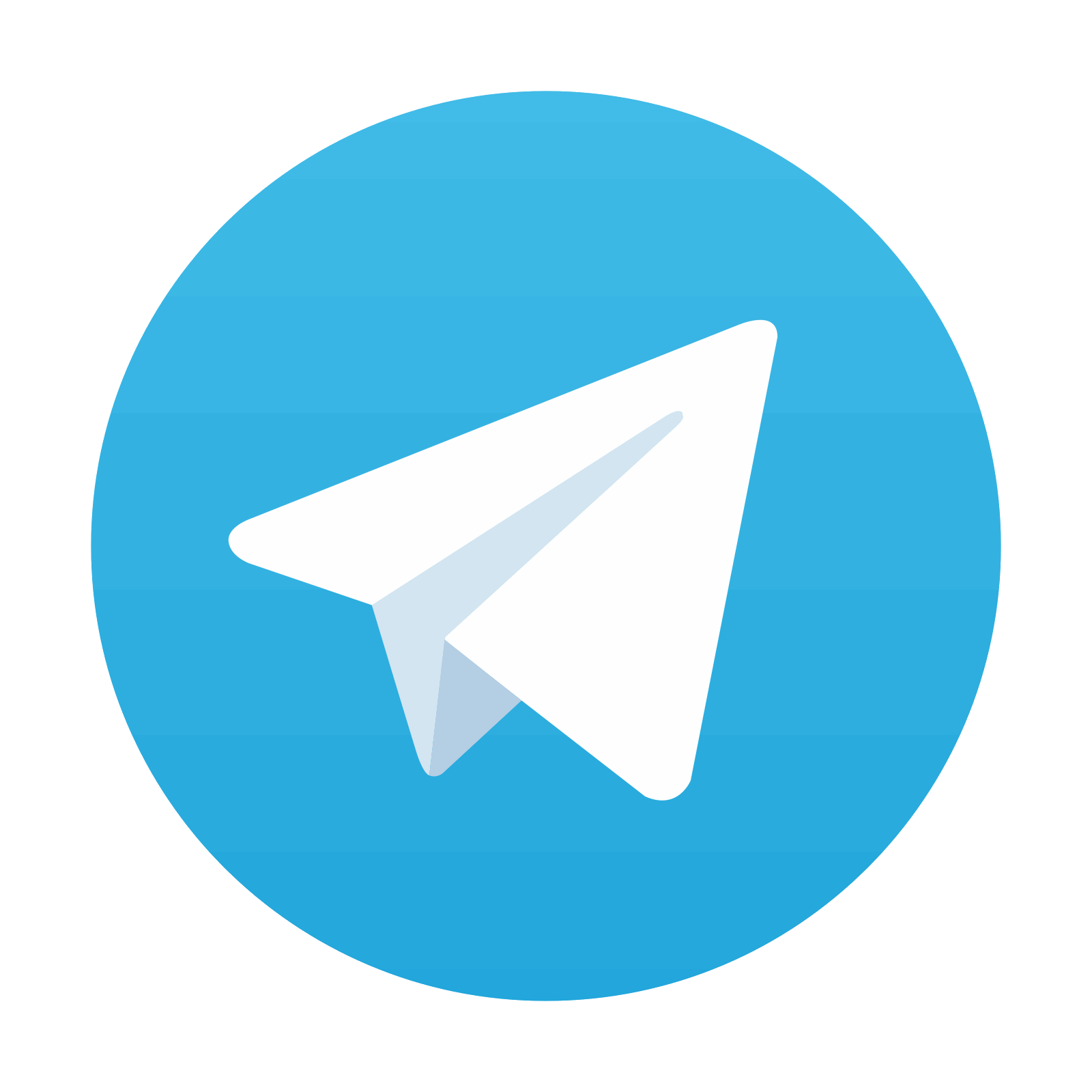
Stay updated, free dental videos. Join our Telegram channel

VIDEdental - Online dental courses
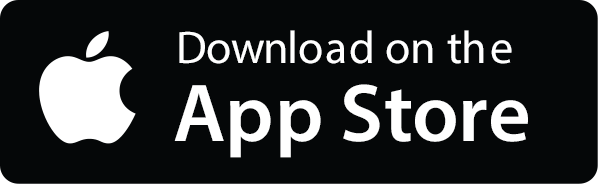
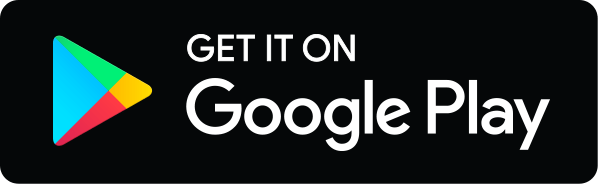