Abstract
Objectives
The phenomena occurring during zirconia frameworks veneering process are not yet fully understood. In particular the study of zirconia behavior at the interface with the veneer remains a challenge. However this interface has been reported to act on residual stress in the veneering ceramic, which plays a significant role in clinical failures such as chipping. The objective of this study was thus to investigate the veneer–zirconia interface using a recent 3D-analysis tool and to confront these observations to residual stress measurements in the veneering ceramic.
Methods
Two cross-sectioned bilayered disc samples (veneer on zirconia), exhibiting different residual stress profiles in the veneering ceramic, were investigated using 2D and 3D imaging (respectively Scanning Electron Microscopy (SEM) and Focused Ion Beam nanotomography (FIB-nt), associated with chemical analysis by Energy Dispersive X-ray Spectroscopy (EDS).
Results
The observations did not reveal any structural change in the bulk of zirconia layer of both samples. However the presence of structural alterations and sub-surface microcracks were highlighted in the first micrometer of zirconia surface, exclusively for the sample exhibiting interior tensile stress in the veneering ceramic. No interdiffusion phenomena were observed.
Significance
FIB nanotomography was proven to be a powerful technique to study the veneer–zirconia interface. The determination of the origin and the nature of zirconia alterations need to be further studied. The results of the present study support the hypothesis that zirconia surface property changes could be involved in the development of tensile stress in the veneering ceramic, increasing the risk of chipping.
1
Introduction
First used as orthopedic implants in the nineties, zirconia was put on the market for use as a framework in prosthodontics about 10 years ago, concurrently to the generalization of Computer-Aided-Design (CAD) and Computer-Aided-Manufacturing (CAM) processes. Because of its biocompatibility, strength and optical properties, it was considered to be a good alternative to metal-based restorations. Unfortunately clinical studies report significant short-term clinical failures of zirconia-based restorations due to cohesive fractures in the bulk of the veneering ceramic (chipping). The origin of this problem, which is less reported with porcelain-fused-to-metal (PFM) restorations, is not yet fully understood .
Partially stabilized Yttria-tetragonal-zirconia-polycrystal (Y-TZP) is a very complex and sensitive material, particularly in regards to the tetragonal to monoclinic (t–m) transformation. The undesired destabilization of the tetragonal (t) phase toward the monoclinic (m) under certain conditions represents the Achilles heel of zirconia . In another hand the veneering concept of zirconia frameworks with feldspathic glass ceramics is empirical and recommended procedures may vary from one manufacturer to another. The sandblasting procedure, which is classically performed with metal frameworks to promote micromechanical adherence, is currently not recommended due to the risk of t–m transformation. However the chemical bond between feldspathic ceramic and zirconia, which are both composed of oxides, is supposed to be effective , even if the interdiffusion of oxides and dopants has been suspected to destabilize the zirconia microstructure . Depending on the system, a thin “liner” material can be fired on the framework as an intermediate layer with the veneering ceramic, similarly to opaque used for PFM restorations. The liner is a partially opaque feldspathic ceramic, it provides some chroma and fluorescence and may also assure wetting of the framework. The properties of veneering ceramics developed for zirconia-based restorations were copied and pasted from veneering ceramics designed for the PFM concept. They were adapted to zirconia frameworks performing Coefficient of linear Thermal Expansion (CTE) measurements and thermal shock testing . Indeed, the CTE mismatch between the bulk materials was designed to mimic the ceramo-metal CTE mismatch, with the CTE of veneering ceramics adapted to be slightly lower than the zirconia. Based on the principle that compressive stress improves the mechanical behavior of the veneering ceramic, this approach was intended to develop residual compressive stress within the veneer during the cooling process . Lately some manufacturers introduced slow cooling procedures of the veneering ceramic to induce some additional residual compressive stress development and to reduce veneer fracture. Recently residual stress profiles were measured in veneering ceramic layered either on metal or zirconia disk frameworks . It was shown that zirconia-based samples behave differently than metal-based samples. As expected metal-based samples exhibited exclusively compressive stress, either in surface or in depth of the veneer layer, while, surprisingly, zirconia-based samples exhibited frequently tensile stress in the veneering ceramic lying near the framework. The presence of interior tensile stress was related to slow cooling rate and to high veneer/framework thickness ratio. The hypothesis of a volume increase of zirconia grains at the interface, due to a t–m phase transformation following the veneer firing process, was proposed to explain these findings . The possible occurrence of a t–m transformation of zirconia during the veneering process was supported by the SEM and X-Ray Diffraction (XRD) observations of Tholey et al., who showed, after removing the veneer with hydrofluoric acid, sub-grain faceting which they attributed to the transformation of the grains toward the monoclinic symmetry at the zirconia–veneer interface . On the other side, a recent confocal Raman microscopic analysis of the interface did not reveal the presence of monoclinic phase , but highlighted a 2 μm-deep silicon–zirconium interdiffusion zone.
The analysis of the veneer–zirconia interface is complex. Most structural analysis techniques (X-ray diffraction, Raman, SEM, Atomic Force Microscopy) are influenced by sample preparation, particularly sectioning and polishing, that can induce microcracks and zirconia structure alteration. Moreover, because of the high resolution needed, interface structural and chemical characterization is a challenge, requiring information from the combination of different techniques.
Focused ion beam nanotomography (FIB-nt) is a recent powerful technique that allows 3D volume reconstruction by electron microscopy, on the sub-100-nm scale. As such, FIB-nt fills the gap between classical tomography methods that operate in the millimeter to micrometer range (e.g. X-ray absorption tomography) and transmission microscopy methods with nanometer resolution (TEM, TXM) . The FIB mills the material through a serial slicing procedure, allowing sequential SEM imaging and 3D reconstruction. This technique has recently been applied to adhesive-dentin interfaces . Moreover the ion beam has also recently been used to mill metal–ceramic and zirconia–ceramic bilayers, showing that it does not alter the zirconia structure .
The first objective of this study was to show the potential of FIB-nt to investigate the veneer–zirconia interface. Chemical analysis at the interface was also performed using energy dispersive X-ray spectroscopy (EDS). A second objective was to confront microscopic features to previous residual stress measurements in the veneering ceramic. On the basis of previous results , two samples with opposite in-depth residual stress profiles in the veneering ceramic were produced and characterized.
2
Materials and methods
2.1
Specimen preparation
Two Y-TZP core disc were cut out of a pre-sintered Vita In-Ceram YZ blocks (Vita Zahnfabrik, Bad Säckingen, Germany), were rounded by polishing, and densely sintered at 1530 °C for 120 min with heating rate 10 °C/min (Zircomat furnace, Vita Zahnfabrik, Bad Säckingen, Germany). The sintered Y-TZP discs, 20 mm diameter, were quenched and sequentially ground with 180-grit and 500-grit silicon carbide discs (Struers LabPol polishing machine, Copenhagen, Denmark) either to a 0.70 mm ± 0.02 mm thickness or to a 1 mm ± 0.02 mm thickness. The Y-TZP discs were exposed to a “regeneration firing”, which is a final thermal treatment of the core to reverse any phase transformation in the zirconia that may occur because of the grinding procedures. The monoclinic fraction after the regeneration was undetectable by XRD, i.e. below 1%.
The Y-TZP disc samples were veneered following standard dental laboratory procedures and manufacturer’s recommendations. A thin coat of Vita VM 9 Effect Bonder was applied and fired on the surface to be veneered. Then, Vita VM9 feldspar veneering ceramic (shade 3M2) (Vita Zahnfabrik, Bad Säckingen, Germany) was progressively layered on the effect bonder. A Vita Vacumat 4000 Premium furnace (Vita Zahnfabrik, Bad Säckingen, Germany) was used for all firing procedures. Three layers of dentin ceramic were successively fired. In comparison with manufacturer recommendations, the firing temperature was maintained six minutes in place of one minute in order to reach 900 °C within the framework. Samples were sequentially ground with 180-grit and 500-grit silicon carbide discs to a veneer thickness of 2 mm ± 0.02 mm for the 1 mm-thick zirconia framework (VZr10), and of 1.50 ± 0.02 mm for the 0.7 mm-thick zirconia framework (VZr07). After final polishing, the specimens were exposed to a last firing cycle. This last firing cycle restored the residual stress profile through the veneering ceramic thickness. Two different cooling schedules were followed. VZr10 was tempered from 900 °C to room temperature by opening the furnace door, as classically done in dental laboratories, and removed from the mesh-tray at 200 °C (Classic Cooling, CC). This schedule was the one used during the veneering layering process. VZr07 was cooled at 2 °C/min in a special furnace (Carbolite LMF 12/2, Carbolite, Hope Valley, UK), from 900 °C to room temperature (Slow Cooling, SC). All firing schedules are summarized in Table 1 . It is to note that the framework and veneer thickness together with cooling rates for both samples were chosen so as to produce final samples with the largest difference in interior stresses .
Starting T (°C) | Pre-drying t (min), closing t | Heating rate (°C/min) | Heating t (min) | Firing T (°C) | Holding t (min) | Vacuum holding t (min) | Slow cooling ending T (°C) | Slow cooling rate (°C/min) | |
---|---|---|---|---|---|---|---|---|---|
Y-TZP core regeneration firing | 500 | – | 100 | 5 | 1000 | 15 | – | ||
Vita VM9 Effect bonder | 500 | 6 | 75 | 6 | 950 | 1 | 6 | ||
Vita VM9 Dentin | 500 | 6 | 55 | 7.27 | 910 | 4 | 7.27 | ||
Last firing cycle | |||||||||
Classic cooling (CC) | 600 | 8 | 50 | 6 | 900 | 6 | 6 | ||
Slow cooling (SC) | Room t ° | 10 | 900 | 6 | Room t ° | 2 |
2.2
Residual stress measurement
Residual stress profiles were measured using the hole-drilling method, following protocols described previously . For the rosette size used, the hole-drilling method can measure residual stresses to depths to 1.2 mm.
2.3
SEM observations and FIB nanotomography
After residual stress measurement procedure, samples were sectioned in the middle with a water-cooled diamond saw (600 μm thick, Struers, Germany), and embedded in epoxy resin. The cross sections of the samples were sequentially ground with 40-, 20-, 10 μm diamond discs and polished with 7-, 3-, and 1 μm diamond pastes, in order to expose the interface between the veneering ceramic and the zirconia framework. A final polishing was performed with colloidal silica on a VibroMet (Buehler, US). Such a technique is often used to remove minor deformation remaining after mechanical preparation.
Each sample was preliminary observed in Scanning Electron Microscopy (SEM) in order to gain some information on microstructures (Supra 55VP; Carl Zeiss Microscopy GmbH, Oberkochen, Germany).
This instrument is equipped with a thermal field emission gun providing a combination of high brightness and high resolution. One other feature of this microscope is the ability to image samples at low accelerating voltage, allowing observation of nonconductive samples without the usual requirement of a metalized surface coating.
So, SEM imaging was done in high vacuum with a standard Everhart-Thornley secondary electron detector and with an accelerating voltage of 1 kV, without any coating.
FIB/SEM imaging was performed using a workstation (NVision 40; Carl Zeiss Microscopy GmbH, Oberkochen, Germany) combining a SIINT zeta FIB column (Seiko Instruments Inc. NanoTechnology, Japan) with a Zeiss Gemini column.
For FIB-nt experiments, the analysed sample was glued with a silver paint onto an aluminum stub. Then, to make the surface electrically conductive, the samples were coated with a carbon layer of about 30 nm by thermal evaporation (EM SCD500, Leica Microsystems GmbH, Germany); indeed, even if the imaging phases are conducted at low voltage, the cutting phases (at higher voltage) make this coating necessary for a more comfortable and precise observation.
Imaging of 2D slices was performed using backscattered electrons (BSE) with the so-called Energy and angle selective BSE (EsB) Detector. This annular scintillation detector is coaxial with the beam. In front of the entry system of the EsB detector, is an energy-filtering grid integrated into the electron optical detection system that can be adjusted in its retarding potential from 0 to 1500 volts. This grid enables only BSE with energies greater than the grid energy to be detected. The FIB-nt experiments were conducted with an accelerating voltage of 1 kV and a grid potential set to 700 V. Such conditions were chosen to fit a cubic voxel side of 10 nm. FIB milling parameters were adjusted to 30 kV and 300 pA to avoid milling-induced transformation.
3D reconstructions from the 2D slides were fully carried out using the software Fiji (Fiji Is Just ImageJ), which is an image-processing package based on the open source project ImageJ ( http://fiji.sc/wiki/index.php/Fiji ).
FIB-nt was performed to confirm SEM observations in the bulk of VZR10 zirconia framework and at the veneer–zirconia interface of VZr07.
2.4
Energy dispersive X-ray spectroscopy (EDS)
X-ray microanalysis was performed by an Energy-Dispersive X-ray System (EDS) with an X-max 80 mm 2 Silicon Drift Detector (Oxford Instruments NanoAnalyis, High Wycombe, UK). In combination with SEM, this technique enables the determination of the local chemical composition (of a region about 1 μm in depth) provided that the elements are in sufficient concentration (starting from some fractions of the percent) and that they are sufficiently heavy (elements heavier than Boron).
EDS spectra were obtained with an accelerating voltage of 10 keV, a process time of 4 μs and a dead time of about 30%.
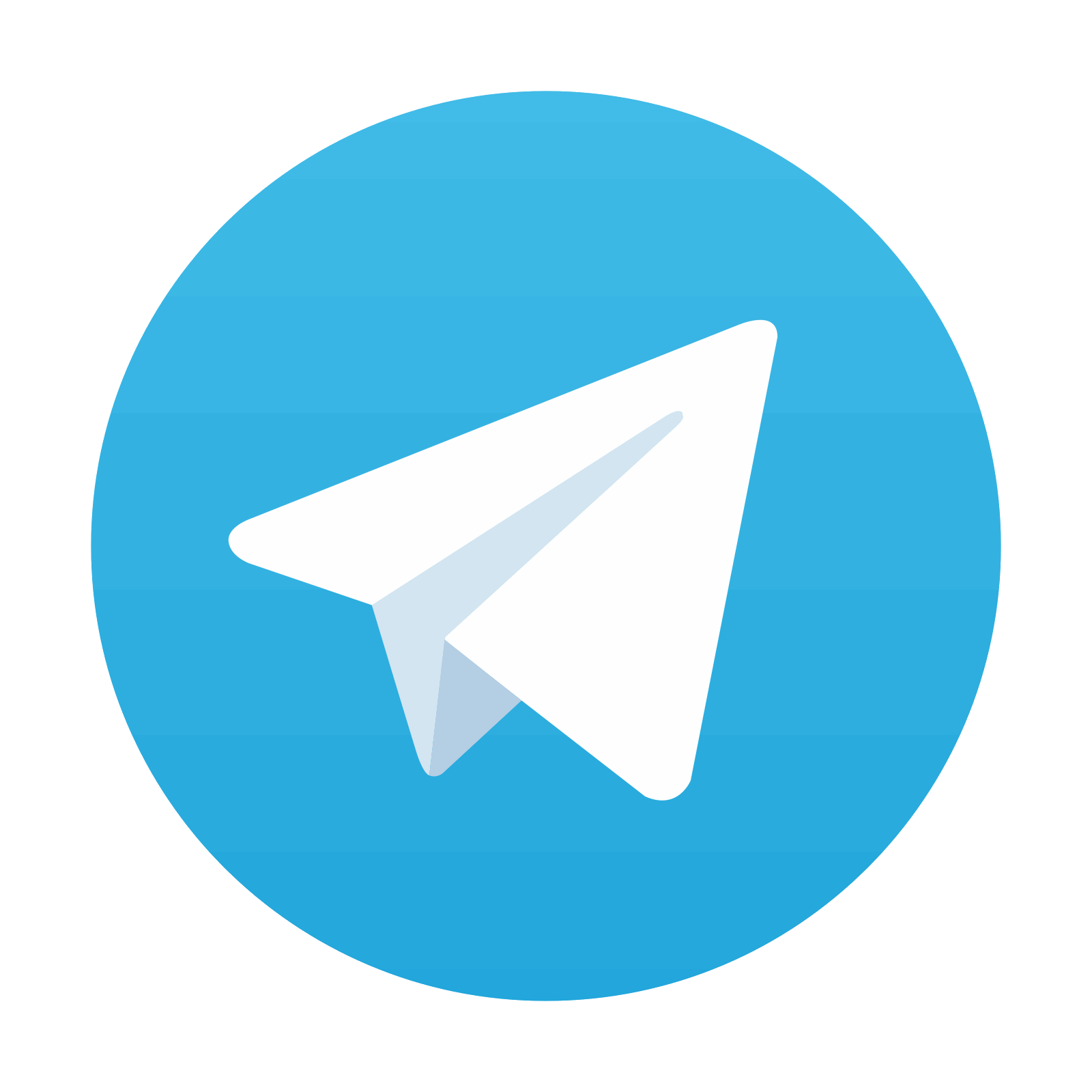
Stay updated, free dental videos. Join our Telegram channel

VIDEdental - Online dental courses
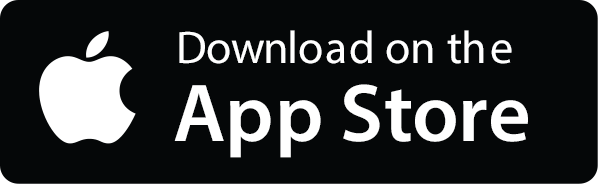
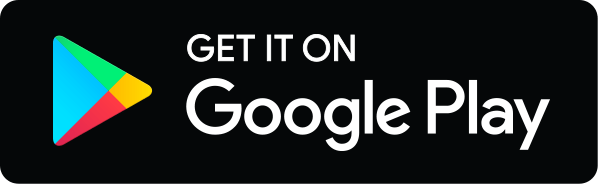