More Polymers
Any treatment of the subject of dental materials naturally emphasizes the ‘most important,’ whether this is because they are used in great bulk, are the basis of a common procedure, or are critical in some sense to the success or quality of outcome of a process. However there is diverse group of auxiliary or adjunct materials whose properties are no less significant, but whose routine or everyday nature may obscure that significance. Some of these have already been dealt with. It is the purpose of this chapter to outline the chemistry and mechanical consequences for a group of such materials which are polymeric.
Rubber dam and gutta percha are closely related chemically, but the marked differences in properties are traced to a simple difference in the structure of the polymer chain. Such structural differences also underlie the great variety of properties that may be found for polysaccharides: paper, starch and related materials are also discussed in those terms.
The polypeptides are a major group of compounds in the sense of biological chemistry, but the mechanical properties of these are also controlled by the details of chain structure. The major use of such materials in the present context is for surgical sutures. Here, the compromise includes considering biocompatibility and resorbability. These factors can also be addressed through synthetic suture materials, and an example is discussed.
The design, selection and handling of materials for use in and around dentistry is essentially based on structure – property relationships. This applies even to ‘minor’ materials that may be overlooked. The ideas of earlier chapters are therefore brought to bear to explain those relationships. It is no less important to understand these materials than it is the others for competent and comprehending use in the pursuit of better dentistry.
Polymers exhibit a very wide range of properties, depending on the chemical nature and structure of the repeating units. The major kinds in use in dentistry have already been discussed, but there remain a number of polymers with specialized but miscellaneous applications. They are of a kind in that they illustrate the links between structure and function as well as the reasons for their selection. However, they have generally received little attention in this field, although of quite different types to those used for impressions, prostheses and restorations.
§1 Polyisoprene
Isoprene or, more formally, 2-methyl-1,3-butadiene (Fig. 1.1) is the notional starting point for an enormously wide range of natural products, the terpenes, which range from essential oils (such as that of ginger) to vitamin A1 and the steroids. The present interest lies in its naturally-produced high polymers: (natural) rubber,1also known as caoutchouc, and gutta percha.2These two differ only in their stereochemistry, that is the relative configuration3 of successive units of the polymer chain. Balata (‘rubber’) 4is similar to gutta percha. (Notice that there is no polymerization reaction involved in any dental context – this has been done by the plant.)
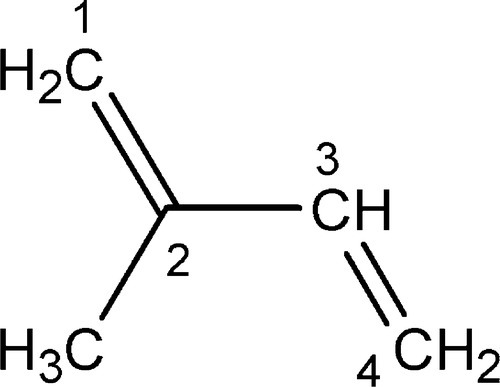
•1.1 Structure comparisons
Rubber, as is used in the manufacture of the elastic bands of orthodontics or rubber dam, is essentially pure cis-1,4-polyisoprene (Fig. 1.2, top), whereas gutta percha, as used in root canal filling points, is nearly pure trans-1,4-polyisoprene (Fig. 1.2). The non-equivalence of these structures derives, of course, from the lack of rotation about the double bond. The stereochemical effect on properties is profound: fresh pure rubber (molecular weight, MW ~ 106) is soft, highly flexible and tacky; fresh pure gutta percha (MW ~ 3 x 104), however, is relatively tough, hard and horn-like, showing comparatively little flexibility.
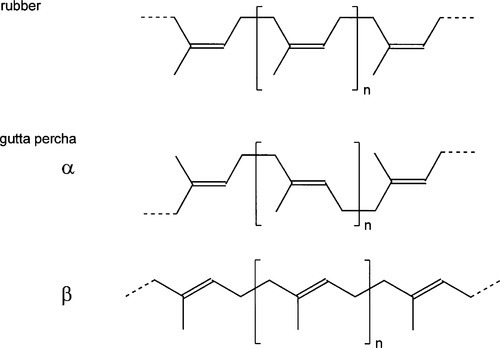
Fresh rubber is normally a gum with little tendency to crystallize but with a ‘melting point’ of about 27 °C, when it becomes very sticky. Gutta percha, on the other hand, is usually about 60% crystalline, 40% amorphous. It has a ‘melting point’, when it also starts to decompose, of about 100 °C. Both polymers have the possibility of free rotation about the -CH2-CH2– bond, but in gutta percha this leads to two distinct crystal forms. At high temperature the chains of gutta percha tend to be in the extended conformation3 in the amorphous, liquid-like state. Rapid cooling, as might be expected, tends to preserve this more extended form in the crystalline β-phase (Fig. 1.2). The more stable and slightly more dense α-phase is formed slowly from the β-phase on slow cooling. The transition from β- to α-phase depends on thermal history, time and temperature, as it relies on the availability of the activation energy for rotation of chain segments to change the conformation; yet another case of kinetic limitation. The density change due to this transformation would tend to spoil the seal in a filled root canal.
•1.2 Gutta percha
Clinical use of gutta percha requires a compromise between the rigidity of the natural product and the mouldability of the plasticized material. Waxes are apparently the additives used to achieve the latter property, while about 80 mass% (~ 42 vol%) zinc oxide is used to control the viscous component of its viscoelastic nature, although this filler would also confer the radio-opacity valuable in identifying the extent, or even the presence, of a filled root canal. Temperature is therefore an important aspect of the use of gutta percha, and moulding to the root canal is thus possible with warmed instruments. Indeed, this occurs slowly at body temperature against soft tissue when it is used as a “functional” impression material in cleft palate patients. Some thermal shrinkage is nevertheless to be expected and this must jeopardize any seal formed in a canal using heat. Since the adaptation obtained would depend on flow, much elastic recovery would also be expected under normal clinical ‘compaction’ procedures. Consequently, some sealing cement is definitely required.
Gutta percha dissolves in, and is also plasticized by, a good many solvents: chloroform and eucalyptol (the chief constituent of eucalyptus oil, Fig. 1.3) are typical. These have been used to soften the surface of gutta percha points to allow better adaptation, although such improvement is necessarily transitory. When solvent dissolves in the polymer there must be a volumetric expansion. The shrinkage that must ensue on evaporation or migration of the solvent after placement will prevent any possibility of a seal being maintained.
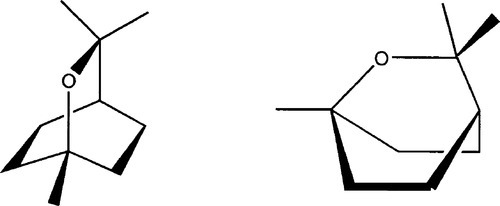
The viscoelasticity of gutta percha has already been mentioned and its slow continuous deformation under stress is of course stress-relaxation. Pure natural rubber is highly elastic, but there is still a sufficient viscous component to allow appreciable stress-relaxation as, again, there is no cross-linking between chains. Indeed, partial crystallization may be induced through the alignment of the molecules (cf. Fig. 3§4.10) and will contribute to permanent deformation. The natural product, then, is not a rubber in the sense of a good elastomer (7§2). There is no three-dimensional network.
•1.3 Vulcanization
Both polyisoprenes have one double bond per chain unit remaining from the original two of the supposed monomer. As in other molecules containing unconjugated organic double bonds, these remain reactive. It was discovered5 that heating natural rubber with sulphur at about 200 °C gave a product that was more elastic and which did not become tacky on warming. Indeed, it stayed flexible at lower temperatures. This reaction between the sulphur and the double bonds results in cross-links being formed. These establish a true elastomeric structure which inhibits crystallization under stress and lowers the glass transition temperature. The process is called vulcanization and employs typically about 1 or 2 mass% sulphur, although now many additives and modifiers are used with a wide range of processing conditions to produce many varied products such as tubing and tyres. Dental applications include rubber dam, where extreme extension is required but, unlike for impression materials, the small remaining viscous component is of little consequence. It is also used as the binder for the abrasive in dental ‘rubber wheels’, but perhaps most significantly in protective gloves. Such vulcanized natural rubber is often called latex rubber, to indicate its natural origin.
Viscous flow stress-relaxation, probably through a bond-exchange mechanism similar to that operating in polysulphide impression material (7§4), accounts for the steady decline in the force applied by latex rubber elastic bands in orthodontics. Thus their frequent replacement is required if the force is to maintained. The relaxation is also aided by the plasticizing effect of absorbed water if they are used intra-orally. Modern elastomeric polymers such as polyurethanes may reduce this flow effect.
Vulcanization was taken to the extreme with very much more sulphur for the denture base material called Vulcanite where the proportions by mass of rubber and sulphur were 2 : 1, very close to the molar weight ratio of the notional monomer unit and sulphur of 68 : 32. Thus, one sulphur atom was provided for each double bond in the polymer. How complete the reaction actually was is not known (and hardly relevant now), but clearly the cross-linking was at least very extensive.
The processing of rubber at high temperature for long periods is not very convenient (and may cause damage) for products such as gloves, and alternative approaches have been developed. To promote the cross-linking of the rubber at temperatures as low as 100 °C, and more quickly, compounds such as zinc dithiocarbamates (Fig. 1.4) are used either directly or as a precursor with zinc oxide, to accelerate the reaction with sulphur. In a reaction whose details are complex and poorly understood,[1] but which is remarkably similar to that of the cross-linking of polysulphide rubber (Fig. 7§4.2), an allylic hydrogen (that is, one that is activated by being on a carbon adjacent to the double bond) is attacked and substituted by the accelerator-sulphur system. The resulting polysulphide side chain can then react with another of its kind to form a cross-link. Subsequent reactions may reduce the number of sulphur atoms to 1 or 2, to stabilize the structure. Many more reactions and types of cross-link are possible, even those based on direct reaction with the double bond, as occurs in the simple process of heating with sulphur alone.
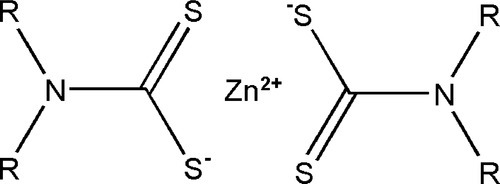
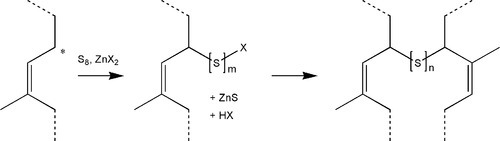
Three factors may represent a hazard to the user. Some accelerators may have carcinogenic potential: they are all meant to be highly reactive, and there will be some residues left in the processed rubber.[2] Secondly, natural latex contains proteins from the parent plant which may be allergenic. A third issue is glove powder: natural latex rubber is tacky, and getting the gloves on is made easier by coating with a powder. Talc (a hydrous magnesium silicate) is no longer used because it causes granulomas if introduced into wounds, but even corn starch-coated gloves (see §2.4) also have problems in surgical contexts,[3] where powder-free gloves are recommended. The dust from these gloves easily becomes airborne and so is respirable, which carries both allergenic proteins and vulcanization accelerators into the lungs. There is yet another problem with latex gloves containing accelerator residues – interference with the setting of addition silicone materials (7§6.3).
To overcome the problem of the tackiness and hydrophobicity of natural latex rubber, and avoid the use of powder, a second layer of a hydrophilic polymer (cf. 5§6.1) may be added.
•1.4 Oxidation
Ordinary vulcanized rubber obviously contains many (if not most) of the original double bonds, while those of gutta percha remain entirely, and these bonds remain vulnerable to attack. In particular, reaction with the oxygen of the air occurs steadily, especially in the presence of ultraviolet light which will produce the π → π* transition (24§6.1) and facilitate the reaction with the ubiquitous diradical. Such oxidation causes cross-linking and other reactions which cause both materials to become brittle, and eventually even to depolymerize. Elastic (natural rubber) bands are well-known to become unserviceably stiff and inelastic with time, and then to be reduced to a sticky mess. Old gutta percha, that is, having been stored for too long, is thus less likely to be serviceable and will be prone to breakage during handling, with consequent difficulties if this occurs in the root canal during placement. In fact, gutta percha in service in a tooth also shows such ageing: an old root filling may be very difficult to retrieve because it has become brittle due to oxidation. The deterioration of elastic (rubber) bands is due to the same reaction, particularly if these are under stress, when the decrease in energy due to stress relaxation as a result of the then available rotation of the bond may be seen to help drive the reaction.
•1.5 Chicle
Another polyisoprene natural product, also collected as a latex, is chicle6. This has both cis– and trans-bonds, in the ratio ~ 1 : 2, which presumably inhibits crystallization. This is used as the basis of chewing gum, of course without cross-linking, and can be used as a means of delivering therapeutic agents to the mouth, although this is only by formulation as a simple mixture so that release is gradual as the mixture is masticated with saliva. This gum, too, shows the effects of oxidation as do gutta percha and natural rubber, although not in any sense that affects its use in practice, although there are implications for discarded gum – a public nuisance in many places.
§2 Polysaccharides
Two polysaccharides have been discussed in the context of impression materials (7§7-9), but another – cellulose – is used in endodontics in the form of absorbent paper points for drying canals and the application of medicaments.[4] This material has many of the general properties to be expected of polysaccharides and therefore can be understood in much the same terms.
•2.1 Cellulose
Cellulose (Fig. 2.1), a major constituent of plant cell walls, is an unbranched condensation polymer of β-D-glucose (Fig. 2.2) using the 1,4- linkage. It consists of fibrils of about 3.5 nm in diameter containing about 40 molecules, each with up to 10,000 sugar residues in wood and 15,000 in cotton. These elementary fibrils are organized into larger bundles, eventually forming the familiar macroscopic fibres that we observe in cotton and paper.
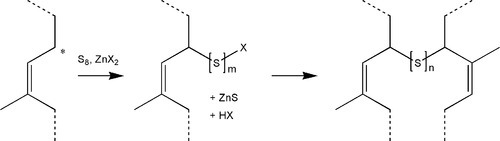
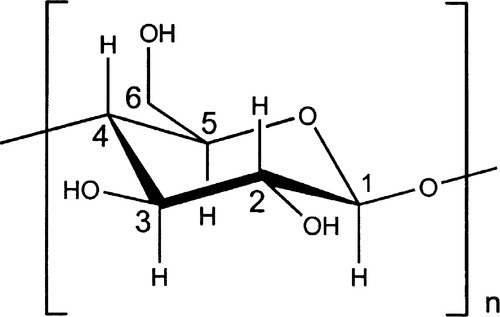
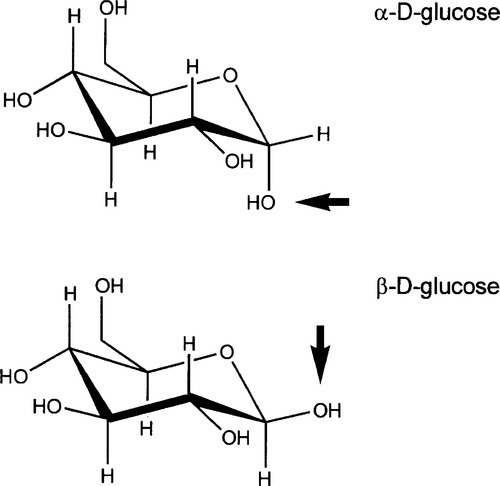
Cellulose is entirely insoluble in water because of the presence of highly organized regions in which the concerted hydrogen bonding is very strong, i.e. there are many bonds close together. However, because of the numerous hydroxyl groups also available in the intervening amorphous regions, which therefore have access to water, the fibrils will absorb large quantities and thus swell somewhat in the process. In this regard the water binding may be compared with that in alginates and agar, although disassembly of fibrils cannot be achieved without using more drastic treatment, such as strong base. In addition, the surface has many ‘exposed’ hydroxyl groups, leading to good adsorption of water. The wetting of such a material is therefore good and the penetration coefficient high (10§2.4), making it ‘absorbent’ and useful in such products as paper towels, the rolled-up slivers called paper ‘points’ for drying prepared root canals, and even the paper used in preparing a porcelain restoration (25§1.2).
•2.2 Paper
Paper relies for its structure and integrity less on fibre entanglement than inter-molecular hydrogen bonding, these bonds forming at contact points between fibres. Water interferes with this very noticeably. Compare the stiffness of a sheet of dry writing paper with that when it is wet, or even when it is merely exposed to a high-humidity atmosphere. Consequently, the wet strength of paper is said to be low. While this may be rather irritating when blowing one’s nose, fibres can easily be shed from endodontic paper points which may cause problems of tissue irritation if they remain, especially at root apices. The wet />
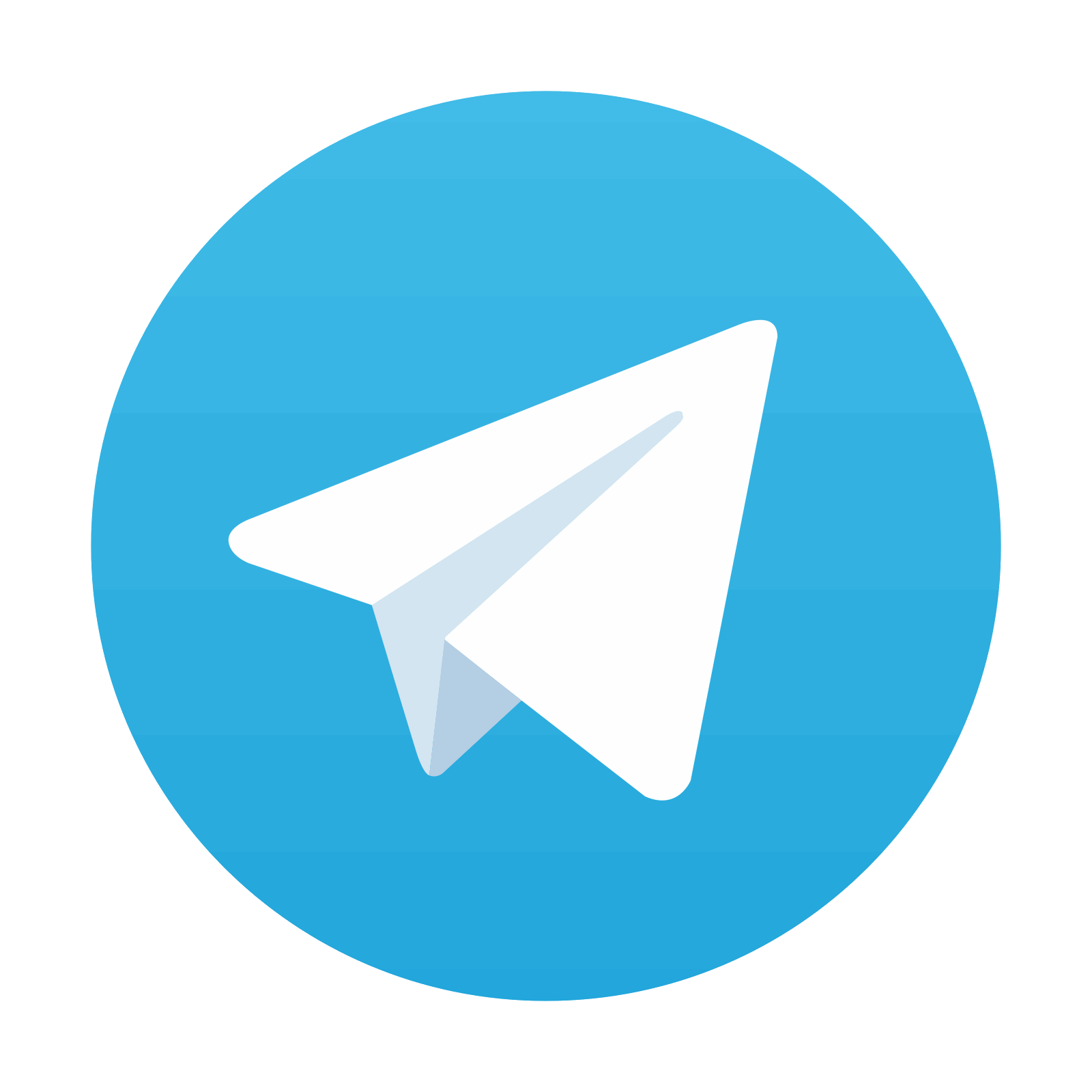
Stay updated, free dental videos. Join our Telegram channel

VIDEdental - Online dental courses
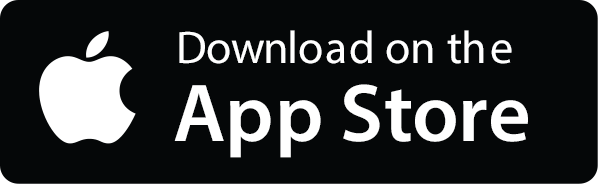
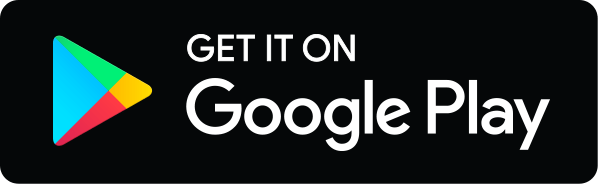