2 Biochemistry and Physiology
1.0 BIOLOGICAL COMPOUNDS
1.1 Sugars and Carbohydrates
A. Carbohydrate terminology
B. Carbohydrate classification—carbohydrates are classified based on the number of simple sugar units they contain. Examples are given in Figure 2-1.
D. Nutritional implications of carbohydrates
1. Monosaccharides are absorbed by a secondary active transport system (Na+ and sugar cotransported) or facilitated diffusion.
1.2 Amino Acids and Proteins
A. Protein terminology
1. Amino acids—building blocks of proteins consisting of a carboxyl group, an amino group, and a distinctive side chain attached to the α carbon. Depending on the side chain, each amino acid has specific characteristics (Figure 2-2).
a. Nonpolar side chains (glycine, alanine, valine, leucine, isoleucine, phenylalanine, tryptophan, methionine, and proline). These promote hydrophobic interactions.
b. Uncharged polar side chains (asparagine, cysteine, glutamine, tyrosine, threonine, serine). These have no charge at neutral pH but may participate in hydrogen bond formation with other compounds.
c. Acidic side chains (aspartic acid, glutamic acid). These have negative charges at neutral pH due to the charge on the carboxyl group.
2. Characteristics of weak acids and weak bases. The Henderson-Hasselbalch equation describes the relationship between pK and pH. Can be used to determine the dissociation of ionizable groups at a given pH.
c. Hydrogen bond—responsible for the secondary structure of proteins. Formed through interactions of the carbonyl oxygens and amide hydrogens that are a part of the peptide.
d. Tertiary structure—the three-dimensional structure of a protein due to side chain interactions of the peptide. May be due to hydrophobic or hydrophilic interactions, hydrogen binding, disulfide bonds, or ionic interactions.
e. Hydrophilic groups—amino acids that are found on the surface of the molecule. Responsible for tertiary structure of proteins.
f. Hydrophobic groups—amino acids that are found in the interior of the molecule. Responsible for tertiary structure of proteins.
g. Disulfide bonds—a covalent link between cysteine groups, which results in a folding of the primary structure. Also responsible for the tertiary structure of protein.
1.3 Lipids
A. Lipid terminology
1. Fatty acids are straight-chained compounds containing an even number of hydrocarbons. They possess a terminal carboxyl group that provides polarity to the molecule. Because of this characteristic, fatty acids are amphipathic (contain both hydrophilic and hydrophobic moieties).
B. Classification of lipids
1. Phospholipids—composed of glycerol or sphingosine, two fatty acids, and phosphoric acid. They are an important component of membranes since they have hydrophobic (fatty acid) and hydrophilic (phosphoric acid) domains. In an aqueous environment, they form bimolecular layers, which are the biological basis of membranes.
a. Phosphoglycerides—composed of glycerol, fatty acids, and phosphate.
(3) PA and ethanolamine = phosphatidylethanolamine or cephalin (important for mitochondrial activity).
(5) Respiratory distress syndrome is caused by the inability to synthesize surfactant (dipalmitoyllecithin).
2. Glycolipids (glycosphingolipids)—composed of carbohydrate, sphingosine, and fatty acid. These compounds are often found on plasma membrane surfaces, especially in nervous tissue. Gangliosides are members of this group and appear to have a role in receptor function.
C. Function of lipids
D. Nutritional implication of lipids
1. Essential fatty acids—cannot be synthesized by mammals and therefore must be obtained from the diet (linoleic and linolenic). Arachidonic acid is synthesized from linoleic acid. Arachidonic acid is a precursor of the eicosanoids, which function as hormone-like molecules in most tissues (Figure 2-4). Prostaglandins, thromboxanes, and leukotrienes are examples of these molecules. If linoleic acid is missing from the diet, arachidonic acid becomes essential.
1.4 Nucleic Acids and Metabolism
A. Nucleic acid terminology (Figure 2-5):
1. Nucleic acids—complex molecules that combine with several different classes of smaller molecules to form either deoxyribonucleic acid or ribonucleic acid.
3. Nucleosides—compounds formed when either a purine or pyrimidine base is linked to a sugar molecule. Examples:
5. Adenonosine triphosphate (ATP)—high-energy nucleotide used as an energy source in numerous biological systems.
B. Classification of nucleic acids
1.5 Nutrients and Minerals
3. Nicotinamide (niacin)
a. Coenzyme form: nicotinamide adenine dinucleotide (NAD) and nicotinamide adenine dinucleotide phosphate (NADP).
7. Folic acid
b. Plays a major role as an intermediate carrier of one-carbon units to appropriate metabolites in the synthesis of nucleic acids and porphyrin compounds.
c. Since folic acid is essential for normal cell division and replication, a deficiency will manifest itself in the most rapidly growing tissues such as in bone marrow and the gastrointestinal tract. Megaloblastic anemia is symptomatic of folic acid deficiency. Maternal deficiency is also associated with neural tube defects in pregnancy.
8. Cyanocobalamine (B12)
9. Ascorbic acid (C)
a. Plays a role in the metabolism of amino acids, in particular the final oxidation of phenylalanine and tyrosine. Also essential for the normal elaboration of the intercellular substance of collagenous and fibrous tissue in animals. As a cofactor in the enzymatic hydroxylation of proline to 4-hydroxyproline, it is required for the formation and maintenance of bone matrix, cartilage, dentin, collagen, and connective tissue in general.
10. Vitamin A
a. Vitamin A plays a role in maintaining the normal structure and function of biological membranes both as components of intracellular organelles or peripheral cell membranes. Epithelial cells also depend upon vitamin A for optimal growth and integrity. The vitamin is also essential for osteoblast and osteoclast activity.
11. Vitamin E
12. Vitamin K
2.0 METABOLISM
2.1 Bioenergetics
A. Terminology
3. Exergonic—the free energy of the products is lower than the free energy of the reactants (δ G < 0). (Net energy is released as a result of the reaction.)
4. Endergonic—the free energy of the products is higher than the free energy of the reactants (δ G > 0). (Net energy is consumed as a result of the reaction.)
5. Equilibrium constant (Keq)—the ratio of the concentration of products to reactants when the reaction reaches equilibrium. The larger the Keq, the more favorable the reaction.
8. Transition state—highest energy arrangement of the atoms that occurs between the reactants and the products.
2.2 Enzymology
D. Enzyme inhibition—numerous substances (including drugs and metabolites) can interfere with enzyme activity. This inhibition may be reversible or irreversible. Three types of reversible inhibitory mechanisms can be identified by enzyme kinetic plots.
2.3 Catabolism
The degradation of large complex molecules into smaller, simple products
A. Carbohydrate catabolism
1. Glycolysis (Figure 2-7)—a series of reactions that convert glucose to pyruvate or lactate. Also produce intermediate for other pathways of metabolism.
d. Important enzymes:
e. Regulators
(3) Phosphofructokinase—inhibited by ATP and citrate, stimulated by AMP and fructose-2, 6-bisphosphate.
3. Tricarboxylic acid (TCA) cycle (Krebs cycle, citric acid cycle) (Figure 2-8):
5. Glycogenolysis—the breakdown of glycogen to form glucose.
a. Important enzymes
B. Lipid catabolism (Figure 2-10):
1. β oxidation—the process by which fatty acids are broken down to acetyl CoA and ultimately utilized as a source of energy in the TCA cycle.
3. Fatty acids are delivered to the mitochondria from cytoplasm by the carnitine shuttle.
4. Important enzymes for β oxidation
5. Regulators
6. Ketone metabolism—under conditions of reduced intake of carbohydrate and increased β oxidation of fatty acids, ketone bodies are formed. Since most of the oxaloacetate is being utilized for gluconeogenesis, acetyl CoA resulting from β oxidation cannot be condensed and further metabolized in the TCA cycle. Acetyl CoA is then conserved by converting it to acetoacetate and β-hydroxybutyrate in the liver.
C. Cholesterol catabolism (Figure 2-11):
D. Protein catabolism
1. Two major enzyme systems are responsible for protein degradation:
2. Pyridoxal phosphate (vitamin B6) is an important coenzyme in transamination reactions using a class of enzymes known as aminotransferases. This is important since the products of this reaction (α keto acids and glutamate) can be used as amino group donors for the synthesis of essential amino acids (glutamate) or enter metabolic pathways for energy metabolism (α keto acids).
3. The liver is the main site for amino acid catabolism where amino groups are removed, converted to urea, and excreted.
5. The carbon groups are further metabolized to pyruvate, acetyl CoA, acetoacetyl CoA, α ketoglutarate, succinyl CoA, fumarate, or oxaloacetate.
6. Peripheral amino acids can be transported to the liver as part of alanine (alanine aminotransferase), glutamate (glutamate aminotransferase), or glutamine.
7. Phenylketonuria, caused by a deficiency of phenylalanine hydroxylase, results in the inability to metabolize phenylalanine. Aspartame, since it contains phenylalanine, is also contraindicated in individuals with this deficiency.
9. Albinism is caused by a defect in tyrosine metabolism and subsequent inability to produce melanin.
10. Since these enzymes are normally intracellular enzymes, elevated plasma levels of aminotransferases indicate damage to tissues containing cells rich in these enzymes (i.e., the liver).
E. Nucleotide catabolism
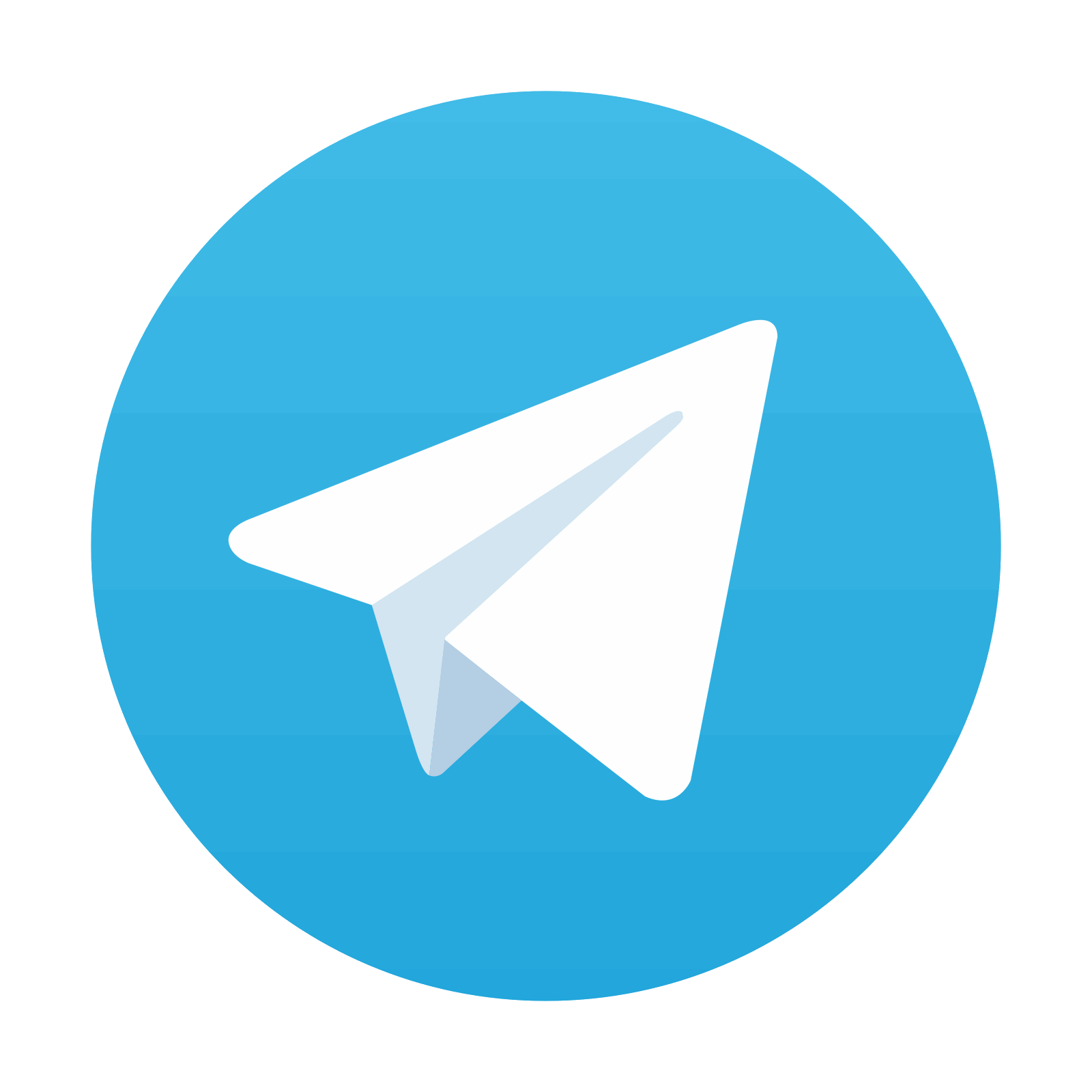
Stay updated, free dental videos. Join our Telegram channel

VIDEdental - Online dental courses
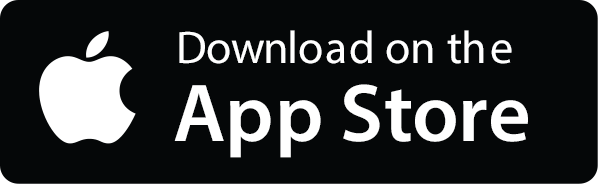
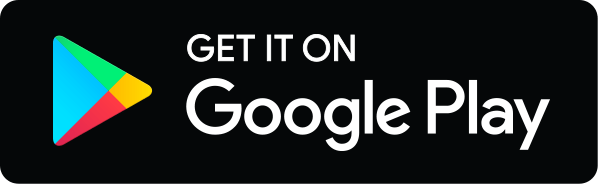