Chapter 15
Optimal Implant Placement in the Esthetic Zone by the Use of Guided Bone Regeneration
Biological Factors Influencing the Reconstruction of Alveolar bone
An absolute prerequisite for implant treatment is the availability of sufficient alveolar bone to support and retain the endosseous implant. Factors such as infection, cystic lesions, tooth/alveolar trauma or congenital tooth agenesis cause a reduction of the alveolar ridge dimensions to a varying degree. With the increasing drive for optimal esthetic outcome of implant treatment, restoring both the hard and soft tissue levels is essential (Fig. 15.1).
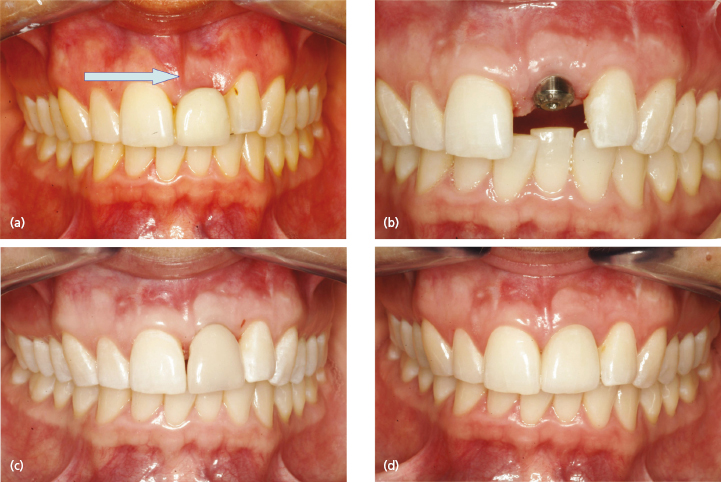
Tooth replacement in the anterior maxilla is a demanding treatment, since the absence of, or poor, preoperative planning, or the choice of an inappropriate treatment approach, can lead to everything from esthetic shortcomings to real disasters.
Esthetic complications can be related to malpositioned implants and the choice of inappropriate prosthetic components. The most critical factors, however, are the anatomic causes that include bone deficiencies in the horizontal or vertical dimensions, and often a combination of the two. This is not infrequently associated with soft tissue defects of the alveolar ridge. Alveolar atrophy and anatomic alterations will have a negative influence on the proper buccal–palatal position of the implant. Malposition of the implant may have effects on the shape, emergence profile, and interproximal contour.
It is important for the clinician to understand that the anatomic contour of the ridge comprises the soft tissue and the underlying supporting bone tissue in all directions. Hence, the soft tissue contour is heavily influenced by the bone anatomy present.
The concept of the so-called biological width has increased the knowledge and understanding of the interaction between the different tissue types and different biomaterial surfaces. In brief, the soft tissue demonstrates relatively constant dimensions in thickness; the peri-implant soft tissue thickness is about 3–4 mm. It is slightly thinner on the buccal aspect and more pronounced at the interproximal areas. The soft tissue is also slightly thicker in the anterior maxillary area in contrast to the posterior region of the mandible, which demonstrates the thinnest portion in the oral cavity.
Due to the relatively constant dimensions of the soft tissue, it is inevitable that the underlying bone structure of the alveolar process plays a key role in the overall esthetic appearance. This is particularly pronounced in the anterior region of the maxilla. This area is also defined as the esthetic zone.
The resorption pattern of the alveolar process following single or multiple tooth loss has been studied in detail and this knowledge is important in the treatment planning of esthetic implant dentistry. Frequently, loss of the buccal bone plate (horizontal resorption) is seen in trauma patients (Fig. 15.1a). This is most pronounced during the first 6 months following the injury. Another important anatomic structure to consider is the interproximal crest height since it plays a significant role in maintaining the peri-implant papillae. Today it is well accepted that vertical resorption of more than 5 mm from the top of the bony crest to the contact point reduces the probability of an intact papilla by approximately 75%. This is particularly the case in situations with multiple tooth loss.
Today’s state-of-the-art esthetic treatment not only involves optimally designed crown and bridge solutions. It also involves restoration of the adjacent tissues (Fig. 15.1). Based on the discussion at the beginning of this chapter, a solid base needs to be created. Thus, reconstruction and correction of bone defects are needed. Numerous methods have been used in an attempt to overcome this problem. Previously, one of the most common methods involved the harvesting and implantation of fresh autogenous bone grafts. However, this involves an extra surgical site, and the morbidity for the patient following such an event should not be ignored. Numerous different types of bone substitute materials have been developed and recent advances are of great interest.
The materials can be divided into three categories:
- Allografts. This group of materials is derived from an individual of the same species and contains no viable cells. The principles for incorporation of allografts follows the same principles as for fresh autogenous bone grafts but the incorporation process probably proceeds more slowly due to the absence of living cells which are both osteoinductive and responsible for triggering the inflammatory process. Frozen or freeze-dried, mineralized or demineralized bone, demineralized dentin, and antigen-extracted allogenic bone (AAA) are all examples of allograft materials.
- Alloplastic grafts. These are developed and derived synthetically and follow the same principles as for allografts, except that the materials contain no proteins and have osteoconductive properties only. Hydroxyapatite (HA) is probably the best known material in this family. Bioactive glass ceramic is another member of this family that has been tested and demonstrates active bone formation. Calcium sulfate (plaster of Paris) has also been evaluated as a grafting material. Recently great interest has been shown in tricalciumphosphate (TCP) materials. This material is osteoconductive and has the capability to be slowly resorbed and eventually replaced by new bone.
- Xenografts. A xenogeneic graft is derived from bone tissue originating from various species. In order to avoid an immunologic response and subsequent rejection after implantation, the proteins have to be eliminated. The osteoinductive capacity of the material therefore disappears and this type of graft material only acts as an osteoinductive scaffold. During the last decade a bovine hydroxyapatite (BHA) (Bio-Oss®, Geistlich, Wolhusen, CH) has been developed and widely used in conjunction with implant treatment. It is by far the best documented material available on the market at present. The material is quite similar to human bone in its configuration and is considered to be osteoconductive (Fig. 15.2).
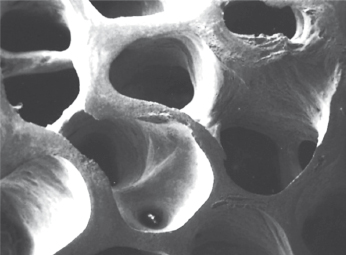
However, there is a discussion about whether BHA is resorbable, or is slowly degraded, or is phagocytable or non-resorbable. Recent findings claim that virtually no resorption occurs even after several years. The biological impact of these findings remains to be fully understood. The fact that BHA particles are incorporated into newly regenerated bone could actually be of an advantage in esthetic situations where the material will act as “reinforcement” of the newly formed bone tissue and thereby prevent early resorption of the regenerated bone.
The Biological Principles of Guided Bone Regeneration
Over the last two decades, the development of the technique of guided bone regeneration (GBR) has had a significant impact on esthetic reconstruction in conjunction with implant therapy. This technique involves the use of physical barrier membranes during the healing phase in order to avoid ingrowth of undesired tissue types into a wound area.
During the 1980s the principle of guided tissue regeneration (GTR) was developed for regenerating periodontal tissues lost as a result of inflammatory periodontal disease. A series of studies documented the possibility of excluding undesirable cells from populating the wound area by means of membrane barriers. This favors the proliferation of defined tissue cells to produce a desired type of tissue (Fig. 15.3).
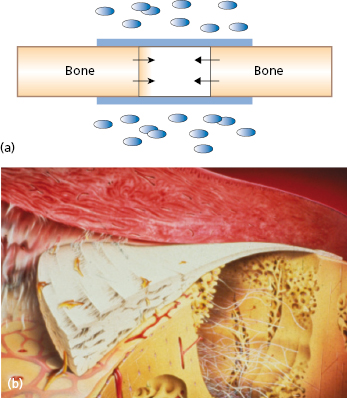
The principle of physical sealing of an anatomic site for improved healing of certain tissue types is by no means new. During the mid-1950s attempts were made for neural regeneration by the use of cellulose acetate filters.
GBR refers more precisely to the goal of the membrane application than GTR. This concept promotes bone formation by protection against an invasion of competing, non-osteogenic tissues. To this end, bone defects are tightly covered by a barrier membrane of defined permeability and excellent biocompatibility.
Experimental studies have proven that certain tissues within the body possess the biologic potential for regeneration if the proper environment is provided during healing. The ultimate goal of GBR is to use a temporary device to provide the necessary environment so the body can use its natural healing potential and regenerate lost and absent tissues.
The efficacy of barrier membranes in conjunction with bone healing and reconstructive therapy is probably the result of a combination of different mechanisms – mechanical, cellular, and molecular. Examples of these are:
- prevention of fibroblast mass action;
- prevention of contact inhibition by heterotopic cell interaction;
- exclusion of cell-derived soluble inhibitory factors;
- local concentration of growth stimulatory factors;
- stimulatory properties of the membrane itself.
The basic studies on GBR have shown that the sequence of healing occurring in regular fracture repair follows the same basic pattern that is found in osseous lesions during GBR therapy. Based on the scientific evidence available, it can be stated that certain conditions must be met for new bone formation to be predictably accomplished by GBR:
- There must be a source of osteogenic cells. Viable bone must be present adjacent to the defect where regeneration is desired.
- An adequate source of vascularity is essential. This supply originates mostly from the adjacent bone surface (Volkmann’s canals and marrow compartment).
- The wound site must remain mechanically stable during healing.
- An appr/>
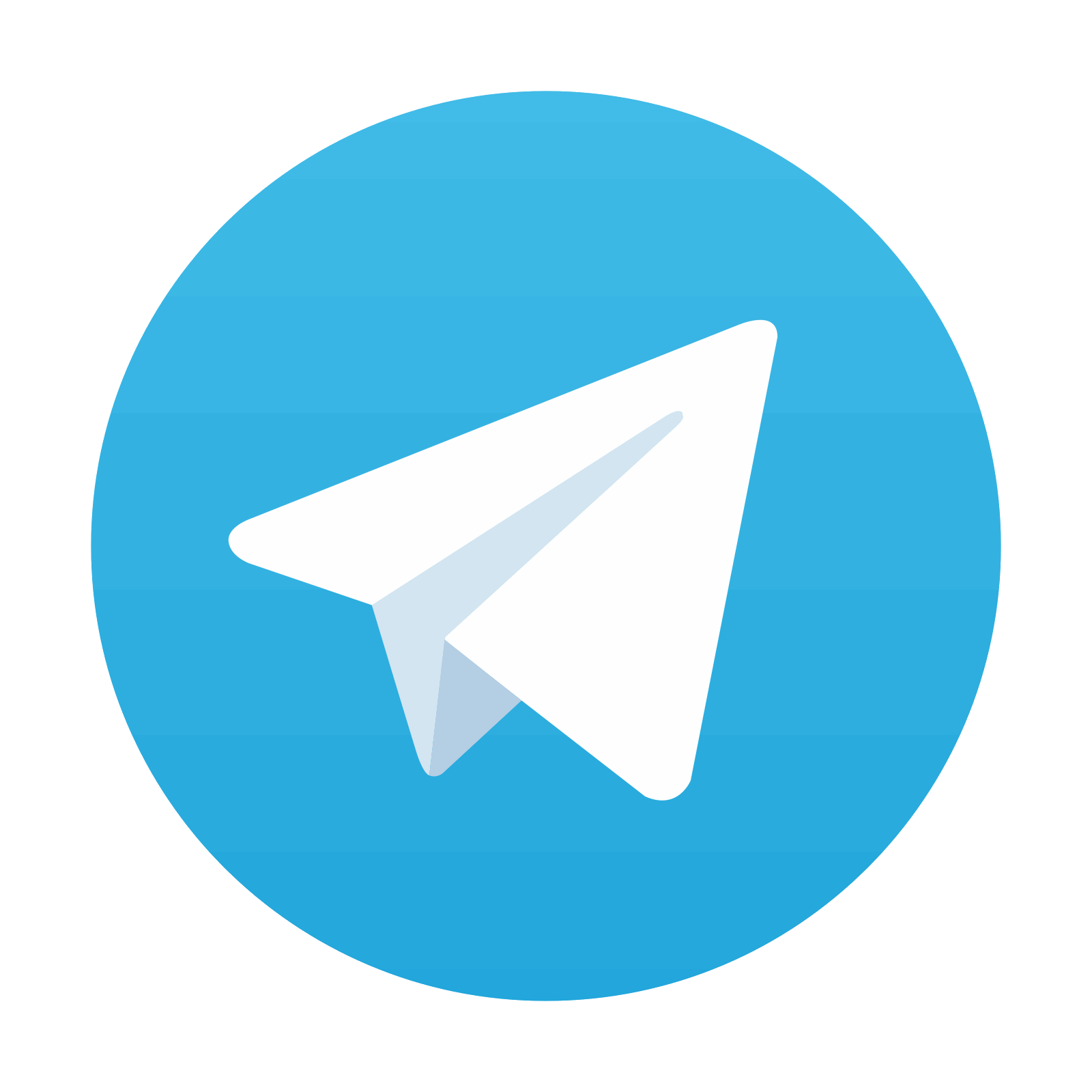
Stay updated, free dental videos. Join our Telegram channel

VIDEdental - Online dental courses
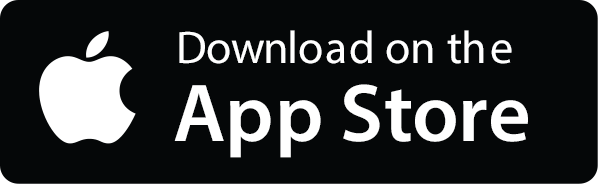
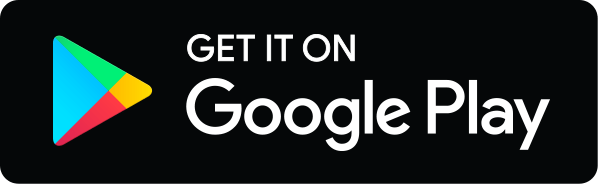